Electrochemistry on the Bench and in the Field
Florika C. Macazo, Ph.D<br>Electrochemistry Product Manager, Bioanalytical Systems, Inc. (BASi), West Lafayette, IN
Electrochemistry is a branch of chemistry involving the study of chemical reactions that produce electrical currents and potentials. For decades, it has been widely used in academic and industrial settings for a myriad of applications in the areas of small molecule sensing, biochemistry, energy storage and conversion, batteries, corrosion, materials research, deposition, and organic electrosynthesis.
An electrochemical cell set-up is composed of either two or three electrodes housed in a small cell or vial. For a 2-electrode set-up, the cell includes a working electrode (WE) and a counter electrode (CE). The potential difference between WE and CE is measured, while the current is equal and opposite at the two electrodes. Likewise, a 3-electrode set-up includes a WE and CE, with the addition of a third electrode — that is, the reference electrode (RE). Here, the potential difference is measured between the WE and RE, while the current is still equal and opposite at the WE and CE. A significant advantage of the latter is its ability to differentiate or decouple the current and potential measurements, which provides better control over the electrochemical measurement.
As shown in Figure 1, various factors contribute to the signal produced in an electrochemical cell. Some of these parameters can be manipulated by the end-user, such as the electrode material and geometry, electrical variables (e.g., potential applied, etc.), and external conditions (e.g., temperature, etc.), while some are more difficult to control, like the mass transfer and solution variables (e.g., diffusion, adsorption, etc.). Regardless, it is essential to tune the parameters that can be controlled to generate reproducible and quantitative electrochemical data. The choice of electrode material, which depends on the measurement performed, and the overall application of the conducted study, can significantly increase the success rate.
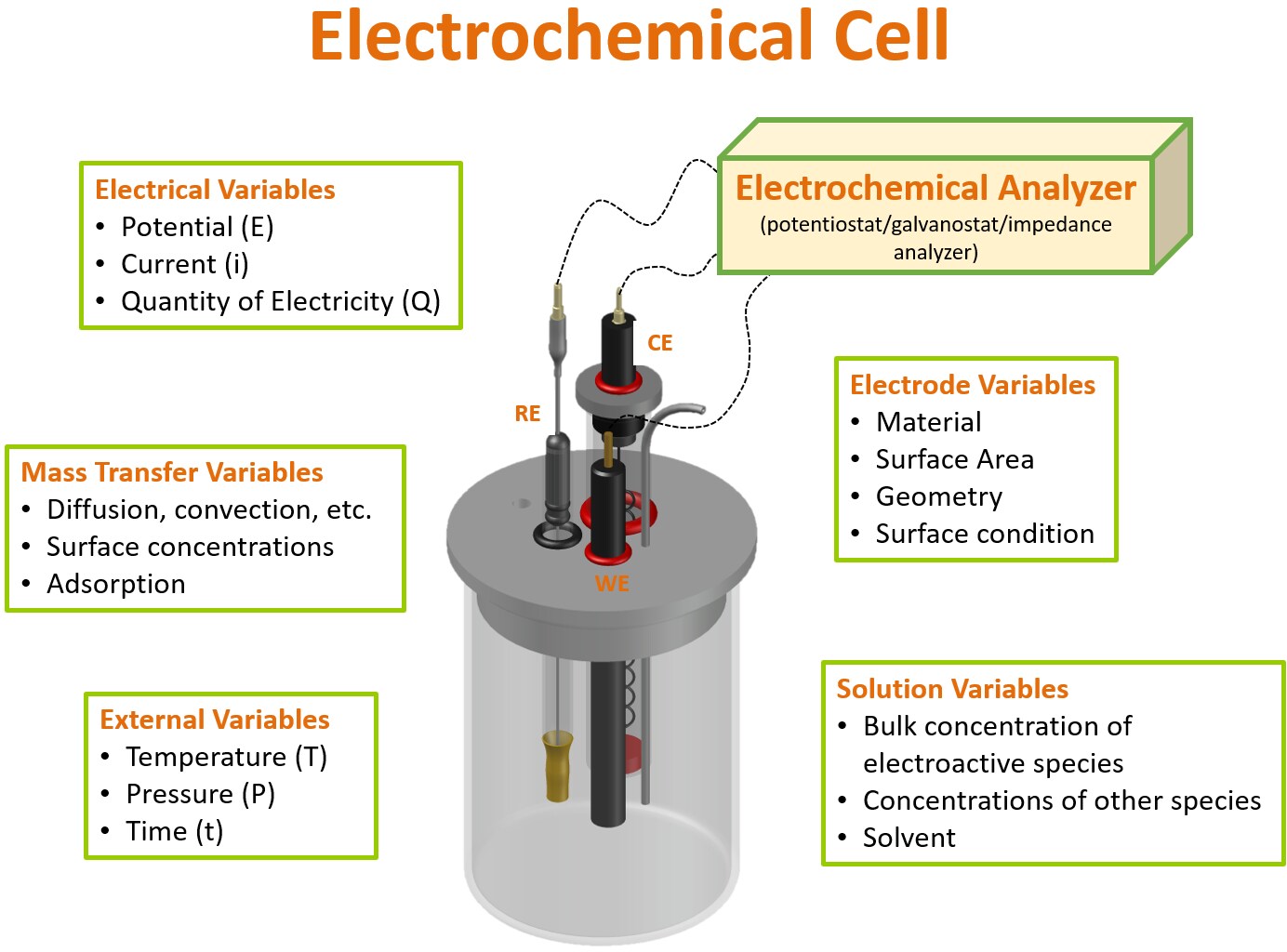
Figure 1. Three-electrode electrochemical set-up and variables contributing to an electrochemical measurement.
Electrodes for Electrochemical Research
Reference Electrodes
Reference electrodes (RE) measure and apply potential between electrodes with a high degree of precision. The use of a reference electrode enables accurate comparison of potential readings between different experiments due to its ability to decouple potential and current measurements in an electrochemical cell. Choosing the right reference electrode for a given experiment is crucial, as it should possess important properties like stable and reproducible potential over time, non-polarizability, low solubility, and reversible coupling that obeys the Nernst equation.
Reference Electrodes for Aqueous Electrochemical Studies
Common reference electrodes used for an aqueous environment include hydrogen, silver/silver chloride (Ag/AgCl), and calomel (Hg/Hg2Cl2) electrodes. The Ag/AgCl reference electrode is the most widely used aqueous reference electrode owing to its stability, ease of use, and convenient construction and disposal. This reference electrode can be assembled using 3M (or saturated) sodium chloride or potassium chloride electrolyte solutions. While this RE provides sufficient properties required for a reference electrode, it does not come without drawbacks. Its disadvantages include liquid junction potentials, contamination of the solvent solution by water, and precipitation at the frit that can cause noise in the electrical readout. Whenever the frit is compromised (e.g., blocked, discolored, broken, chipped, etc.), the reference electrode can be cleaned and regenerated by replacing the CoralPor® frits and electrolyte solution in the reference compartment.
Product Recommendations: |
---|
Reference Electrode for Non-Aqueous Electrochemical Studies
Specific research involves non-aqueous, organic conditions. Since the composition of the solution and concentration of electroactive species present contribute primarily to the electrochemical signal, the use of a compatible, functional reference electrode in this environment is critical to the success of the measurement. While there are not many commercially available reference electrodes for non-aqueous electrochemical studies, the Ag/Ag+ reference electrode has gained traction among researchers in the field. Here, a silver wire makes contact with a silver ion solution, usually provided by AgNO3, AgClO4, or other silver salts in the chosen electrolyte. Often, the electrolyte in the reference compartment is the same as the solution electrolyte. Many studies in scientific literature report the use of various salts, such as tetrabutylammonium perchlorate (TBAP), tetraethylammonium perchlorate (TEAP), tetrabutylammonium hexafluorophosphate and tetrabutylammonium fluoroborate. Typical polar or dipolar aprotic solvents used to dissolve these organic salts include acetonitrile (ACN), dimethylformamide (DMF), dimethylsulfoxide (DMSO) and propylene carbonate (PC). In cases where a salt is not sufficiently soluble in the solvent (e.g., methylene chloride, tetrahydrofuran, etc.), a more polar yet miscible solvent, like acetonitrile, is recommended.
Product Recommendations: |
---|
***This kit contains material required for construction of a non-aqueous silver/silver ion reference electrode. The glass electrode body has a porous CoralPor® frit attached with heat-shrink tubing. The removable Teflon top contains a silver wire and 0.060” gold-plated connecting pin for the cell lead. The kit also contains solid silver nitrate, extra heat-shrink tubing, and extra porous CoralPor® tips. Non-aqueous solvents must be supplied by the user.
Working Electrodes
Working electrodes (WE) provide the conductive surface where the electrochemical reaction occurs. As the potential is applied, the electroactive surface of a given working electrode enables the transfer of electrons to and from the bulk liquid solution, producing a current response which yields an electrochemical readout. The resulting current from the applied potential is equal and opposite at the working and counter electrode surfaces. Selecting the appropriate working electrode varies from application to application, however it must be generally inert, conductive, and provide efficient electron transfer kinetics and thermodynamics to facilitate consistent and highly sensitive electrochemical measurements.
Various materials have been explored and used as working electrodes over the years; however, the most established are comprised of platinum, gold, and glassy carbon. Other materials include copper, nickel, zinc, and silver. It is paramount that the surface of a working electrode is very clean, and has sufficient roughness for the electrochemical reaction to occur. If the working electrode surface is fouled with contaminants (e.g., molecules diffusing from the bulk solution, or a particle stuck in the electrode surface), this dramatically affects the electron transfer kinetics and the current response of redox-active species in the solution, resulting in irreproducible data. In this case, it may be necessary to clean and pretreat the electrode by rinsing it with a copious amount of deionized water and/or methanol to remove adsorbed species. Stubborn debris or organic particulates may require hand polishing with a diamond or alumina suspension applied to a micro-cloth or polishing pad. Always proceed from coarse to fine materials in the polishing progression, with thorough rinsing and changing of pads between each step.
Product REcommendations: |
---|
Counter Electrodes
Counter electrodes (CE) are highly inert, conductive metal electrodes utilized to “counter” or balance the electrochemistry taking place at the working electrode, and provide a source or sink of electrons in the cell. The potential of the counter electrode is determined by the electrochemistry occurring at its surface. In addition to the properties mentioned above, a counter electrode should generally have a larger surface area than the working electrode to avoid overloading the current. To date, materials employed as counter electrodes include platinum of different geometries (e.g., wire, flag, or gauze), graphite rod, stainless steel, gold, and silver.
Product Recommendation: |
---|
Techniques for Electrochemical Research
Electrochemical Methodologies
Since the introduction of electrochemistry in academic and industrial research, several electrochemical techniques have been developed and used. The quantitative data desired dictates the preference for one electrochemical technique over the other, and varies depending on the end-user. Electrochemical measurements are typically obtained via voltammetry, amperometry, coulometry, potentiometry, and impedance spectroscopy (Figure 2), although cyclic voltammetry is the most widely used basic electrochemical technique.
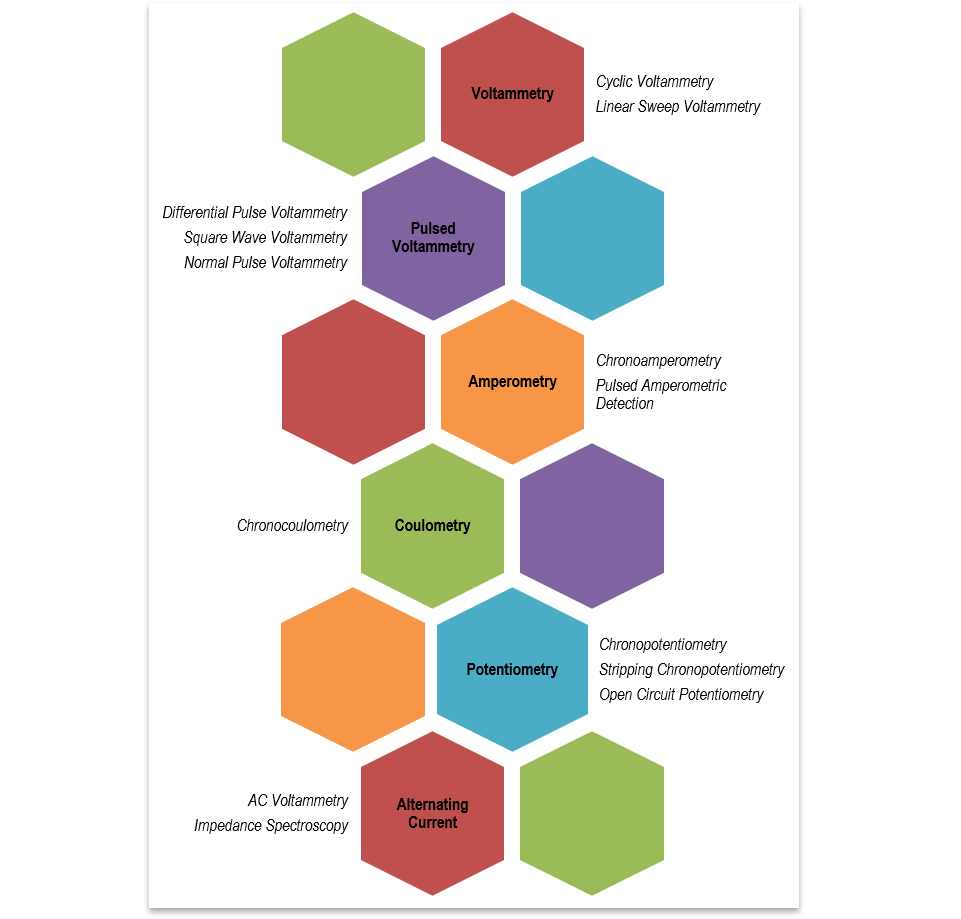
Figure 2.Electrochemical techniques.
Cyclic voltammetry (CV) offers an excellent initial characterization technique as it provides useful information on electron transfer kinetics, thermodynamics, stability, and adsorption of redox-active species in the solution. Below is an example of how CV can be used in the detection of real-world samples, like acetaminophen.
Electrochemistry in the Field: Small Molecule Sensing Using Cyclic Voltammetry
Acetaminophen (N-acetyl-p-aminophenol, APAP), the active ingredient in Tylenol, is a widely used analgesic. One side-effect, though, is that liver and kidney damage may result when administered in large doses. One of the primary methods of metabolism in the liver involves oxidation of the drug. Therefore, it is advantageous to study the oxidation (redox) chemistry of acetaminophen and its metabolites. As a mono-substituted aminophenol, it is electrochemically active. The voltammetric behavior indicates a 2-electron oxidation at moderate potential with significant but not ideal reversibility (Figure 3). By varying the solution pH and scan rate of the experiment, information can be extracted and pieced together to elucidate the mechanism.
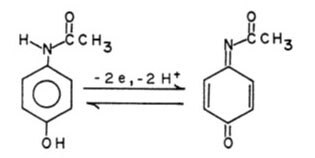
Figure 3. Two-electron, two-proton oxidation of acetaminophen to an electrochemically active intermediate.
This example provides a practical application of how CV is used for the determination of quantitative concentration and mechanistic information. Offering an alternative to more conventional methodologies used in detecting certain small molecule drugs, CV measurements can be used quantitatively to determine an unknown concentration of acetaminophen in an acetaminophen tablet, and qualitatively to demonstrate mechanistic information. In this study, the electrochemical cell was assembled by placing the glassy carbon working electrode (GCE) (BASMF2012-1EA), platinum wire auxiliary electrode (BASMW1032-1EA), and Ag/AgCl reference electrode (BASMF2052-1EA) in the solution to be analyzed. CVs were obtained for five standard solutions to establish a calibration curve (Figure 4), and the absence or presence of saturating concentrations of acetaminophen (Figure 5) to study the electrochemical oxidation of acetaminophen.
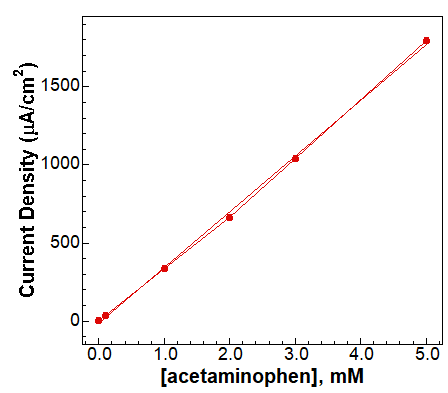
Figure 4. Calibration curve – acetaminophen standards.
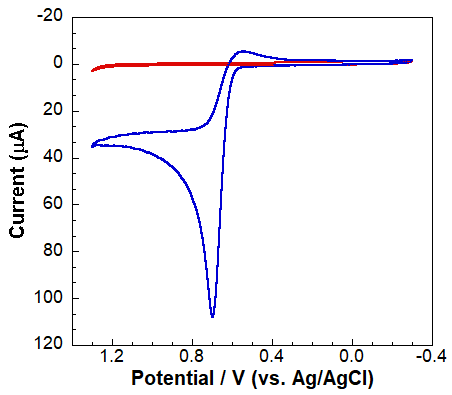
Figure 5.CV of GCE in the absence (red) and presence of acetaminophen (blue).
Figure 5. CV of GCE in the absence (red) and presence of acetaminophen (blue).
Single, reasonably well-defined cathodic and anodic peaks indicate that only two electroactive species are involved. In Figure 5, the anodic wave represents the oxidation of acetaminophen, while the cathodic wave represents the reverse reaction (reduction to acetaminophen). At lower pH’s, the solution is sufficiently acidic to protonate the oxidized species (Figure 3), giving a relatively unstable but electrochemically active intermediate. This intermediate rapidly undergoes hydration to form an electrochemically inactive species, as shown in Figure 5. The absence of a well-defined cathodic current indicates that any intermediate generated by the oxidation rapidly undergoes a chemical reaction to form an electrochemically inactive species. Using the calibration curve (Figure 4) and voltammograms obtained for the unknown (Figure 6, estimated concentrations of the dilute and concentrated unknown acetaminophen tablet are 1.99 ± 0.15 mM and 4.15 ± 1.49 mM, respectively.
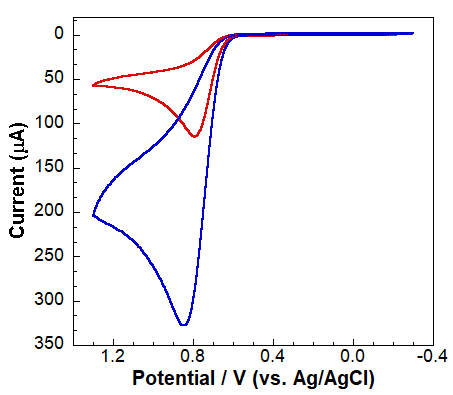
Figure 6. CV of GCE in diluted (red) and concentrated (blue) unknown solutions.
References
Per continuare a leggere, autenticati o crea un account.
Non hai un Account?