Poly(2-oxazoline)s (POx) in Biomedical Applications
Robert Luxenhofer1, Rainer Jordan2
1Functional Polymer Materials, University Würzburg, Röntgenring 11, 97070 Würzburg, Germany, 2Professur, TU Dresden, Mommsenstr. 4, 01069 Dresden, Germany
Material Matters, 2016, 8.3, 70
Introduction
Poly(2-oxazoline)s (POx) can be viewed as conformational isomers of polypeptides. They are synthesized via living cationic ringopening polymerization (LCROP) of 2-oxazolines and were almost simultaneously discovered in 1966 by the groups of Litt, Tomalia, Fukui and Seeliger.1-3 Extensive studies by Saegusa, Kobayashi, R.C. Schulz, Nuyken, Ringsdorf and many others demonstrated the highly living character of the LCROP, and numerous POx homopolymers, block, random, gradient copolymers and functionalized POx were reported.2,4 A recent special issue by Wiley-VCH (Macromol. Rapid Commun. 2012, 33 (19), 1593- 1719) addresses most aspects of the chemistry, properties and applications of POx and related pseudo-polypeptides in several reviews. Here, we give a brief summary of the synthetic possibilities related to POx and potential applications of POx-based materials with a focus on biomaterials and nanomedicine.
Synthetic Possibilities and Physical Properties
The synthetic possibilities of the POx system are outlined in Figure 1. The structural as well as functional variation of POx polymers can be varied by the use of mono- to multi-functional initiators along with the incorporation of functional 2-oxazoline monomers.
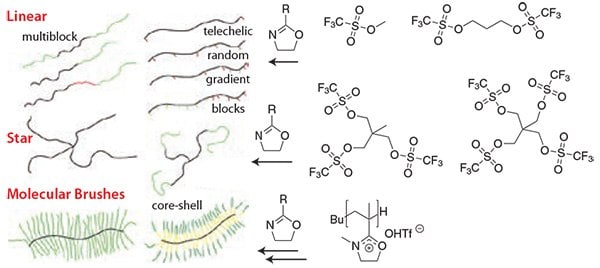
Figure 1.The structural and chemical variability of POx can be realized by the initiator and choice of additional functional monomers. Mono- or difunctional initiators result in linear POx, multifunctional initiators into POx stars, and macroinitiators in well-defined molecular brushes. For the latter, 2-isopropenyl-2-oxazoline is first polymerized by radical or anionic polymerization. Reaction with methyltriflate gives the macroinitiator salts, which react with most 2-oxazolines by LCROP to molecular brushes.
Although promising POx-based systems presented in the early 1990s resulted in initial commercialization, the development of POx lay dormant for almost two decades. Only recently, issues with and limitations of poly(ethylene glycol) (PEG)-related biomaterials triggered an intensive research on POx materials.5,6
The main physical properties of POx are defined by the nature of the side chain residue. In general, POx are amorphous or semi-crystalline polymers. While the melting point is found around 150 °C for most semi-crystalline POx, the glass transition temperature lies between +100 °C and –80 °C, depending on the specific nature of the side chain.7-8
Interestingly, most POx are miscible with a broad variety of other polymers and soluble in various organic solvents. As the POx main chain features a polar tertiary amide group, the entire range from highly hydrophilic to hydrophobic POx can be realized.
Short (< C4) pendant chains result in water soluble polymers while longer aliphatic or aromatic side chains result in hydrophobic polymers (Figure 2). Similar to other water-soluble polymers, aqueous POx solutions exhibit reversible phase-transitions (C2 and C3 side chains) which renders POx as an ideal candidate for the design of “smart polymer materials.” 9,10 In contrast to some other prominent temperature-sensitive polymers, such as poly(Nisopropylacrylamide) (PNIPAM, Product No. 724459), the phase transition of POx solutions occurs with only a minor hysteresis and within a very narrow temperature interval. Variation of the pendant group, as well as copolymerization of hydrophilicand hydrophobic-substituted 2-oxazolines with iso- or n-propylsubstituents (PiPOx, PnPOx), allows a broad adjustment of the cloud point (Tcp) over the entire temperature range (0 to 100 °C) as well as fine-tuning the soluble-to-insoluble transition temperature around human body temperature. As outlined in Figure 2, the introduction of n-alkyl substituents in the pendant chain results in so-called “polysoaps,” meaning that each monomer unit has an amphiphilic motif. Block copolymerization of hydrophilic and hydrophobic 2-oxazolines thus yields polymers of an amphiphilic contrast in the monomer unit as well as in the polymer main chain.
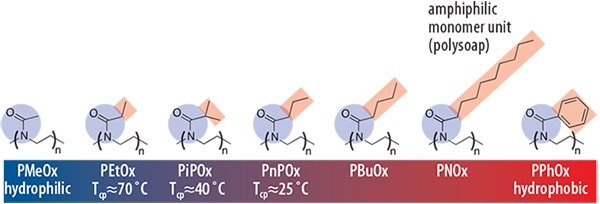
Figure 2.Water solubility of a series of poly(2-oxazoline)s as a function of the polymer pendant group. While poly(2-methyl-2-oxazoline) (PMeOx, Product No. 774189) is highly hydrophilic, poly(2-ethyl-2-oxazoline) (PEtOx, Product No. 773379) exhibits a so-called “cloud point temperature” (Tcp), which varies with architecture, concentration, and molar mass. This is the critical temperature above which the polymer becomes instantaneously water insoluble. The Tcp decreases further to 40 °C for poly(2- iso-propyl-2-oxazoline) (PiPOx) and PnPOx until poly(2-butyl-2-oxazoline) (PBuOx) is the first insoluble POx. Longer alkyl-substituents or aromatic moieties render the polymer hydrophobic but with an amphiphilic motif in the monomer unit (polysoap).
The narrow transition temperature window, as well as the tunability for different target temperatures, creates an interesting comparison to other common polymer amphiphiles including PEG systems (Pluronics). This capability to generate material with tailored properties is also applicable to POx of more complex architectures32 and end groups.11
Lipopolymers
Functionalization by the initiating and/or terminating step can also be performed with lipids as the hydrophobic moiety resulting in so-called “lipopolymers” (Figure 3). In early studies, POx-based lipopolymers were synthesized and studied during the discovery of the well-known “Stealth Effect” of hydrophilic polymers that are conjugated to biomolecules.12,13 The stealth effect refers to a decreased immune response and increased residence time. However, only PEG-based systems became widely known for this property. Later, POx-lipopolymers were employed successfully for the design of stable biomimetic model membranes in the form of liposomes, monolayers at the air-water interface, and as solid supported (polymer-tethered) membranes with a favorable performance of POx in comparison to the PEG-based systems. This was due to the broader chemical variability of POx and its hydrophilic nature, as well as the different interaction potential of POx with incorporated transmembrane proteins.14 Some examples of lipopolymers realized with the POx system are summarized in Figure 3.
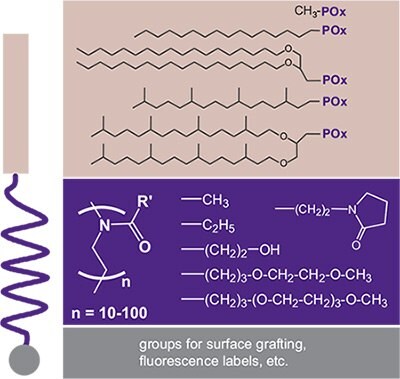
Figure 3.Selection of POx-based lipopolymers with a modulation of the hydrophilic– lipophilic balance through different combinations of n-alkyl or lipid moieties and POx. The variation of the POx pendant groups also allows a modulation of the polymer–water interaction (hydrogen bonding) including oligo(ethylene glycol) (OEG) moieties. The terminal end of the lipopolymers can be further functionalized by surface coupling groups such as silanes (directly using α,ω-aminoalkylsilanes) or by polymer analog reactions after termination with (protected) piperazine (e.g., fluorescence labeling).
Biomedical Applications
Biocompatibility of POx and Issues with PEG
While the early findings on the Stealth Effect triggered the extensive research and clinical application of PEG-based lipopolymers and countless PEGylated conjugates, “POxylated” analogs were forgotten for about 10 years. One reason might be found in the early commercial availability of a broad range of defined PEGs. Recently, further studies on hydrophilic POx (poly(2-methyl-2-oxazoline) (PMeOx) and (poly(2-ethyl-2-oxazoline) (PEtOx, Product No. 773379) in a biomedical context underlined the high potential of this material. Analogous to PEG, PMeOx or PEtOx exhibited very little to no organ uptake (including liver) in mice15 and are essentially not cytotoxic even at rather high doses of administation.16,17 This also includes amphiphilic POx which are premier candidates for micellar drug delivery.18 Moreover, safe administration of POx-based polymer therapeutics was recently demonstrated in non-human primates. Non-specific protein binding is also very low on POx-modified surfaces. The performance is, again, similar to PEG-based systems; however, as PEG is a polyether and, thus, prone to oxidative degradation,19 POx was found to be stable under physiological conditions for a long time and thus probably more suitable for long-term use in animals and humans.5 Considering that PEG has already been extensively investigated, the POx-based biomaterials must prove their suitability as an equal or better alternative. An important factor will be the immunogenicity of POx-based materials. Recently, it was found that PEGylated compounds activate the immune system to a larger extent than originally expected (activation of the complementary system and formation of antibodies).20,21 Consequently, PEGylated compounds are rapidly cleared from the blood if administered repeatedly (anti-PEG immunity).22-24
Polymer Therapeutics: POx in Nanomedicine and Cancer Research
Because of the highly structural and chemical variability, POx are ideally suited for the design of polymer therapeutics of all main types: polymeric micelles for drug-delivery, POx-drug and POxprotein conjugates, and polyplexes.3,6,25 In this contribution we can only spotlight recently developed systems to address the advantages and specific potential of POx. In comparison to the currently used “gold standard” PEG and the related poloxamers, all preclinical data indicate the suitability of the polymer for medical use. Until now, however, POx has not entered clinical trials and are not approved by the U.S. Food and Drug Administration (FDA) as part of a formulation or drug that can be administered to humans. PEtOx, however, are approved as an indirect food additive by FDA.
POxylation: POx-drug and POx-protein Conjugates
Modification of proteins with POx (POxylation), analogous to PEGylation, was pioneered by Saegusa.26 In this early report and since, POx were modified post-polymerization to react with amine moieties of proteins. Proteins such as horseradish-peroxidase, superoxide dismutase (SOD1), albumin, trypsin, granulocyte colony stimulating factor, and others were POxylated.27-29 The majority of this work was dedicated to finding alternatives to PEGylated proteins. Accordingly, hydrophilic POx such as PMeOx and PEtOx were utilized. However, one major limitation of PEGylation of proteins is the decreased mobility of PEGylated proteins across biological barriers. Since we previously showed that the control over POx composition allows for excellent control over polymer endocytosis,16 it was hypothesized that amphiphilic POx will facilitate transport of covalently attached proteins via cellular membranes, similar to some members of the poloxamer family.30-32 Indeed, it was observed that SOD1 modified with amphiphilic POx did exhibit increased neuronal uptake in vitro.28 Decreased concentration of reactive oxygen species (ROS) showed protein delivery in active form. More importantly, it was shown that amphiphilic POx enabled the transport across the blood-brain barrier in vivo.
POx-based Polymeric Micelles for Drug Delivery
Solubilization of hydrophobic drugs with amphiphilic small molecules and polymers is a direct approach to prepare a formulation suitable for parenteral administration. However, low drug concentration in the formulation and poor formulation stability are significant drawbacks typically encountered. The LCROP of POx allows excellent and straightforward tailoring of amphiphilic block copolymers to result in non-ionic polysoaps, and amphiphilic POx block copolymers self-assemble into highly defined and relatively small polymeric micelles as shown in Figure 4. Surprisingly, no drug delivery platform has been developed using this material for a long time. Jeong and co-workers have utilized a block copolymer comprising PEtOx as the hydrophilic block and poly(ε-caprolactone)33 for the solubilization of paclitaxel (PTX). Although the formulation was relatively effective and safe, it appears that this approach has not been developed further. One reason may be that the amount of paclitaxel that could be incorporated was rather low by this approach.
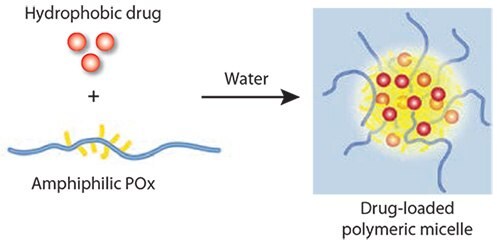
Figure 4.Simple but highly effective solubilization of hydrophobic drugs by amphiphilic POx. After addition of water to polymer–drug films, stable micellar solutions of nearly water-insoluble drugs are obtained.
We recently discovered that block copolymers with poly(2-butyl- 2-oxazoline)s as a hydrophobic block allow the solubilization of very large amounts of hydrophobic drugs, including amphotericin B, paclitaxel, cyclosporine A, 17-AAG and others.18 Not only is the relative amount of the drug in the formulation as high as 50 wt.%, the overall solubility remains very high and stable micellar solutions were observed. Moreover, it is possible to dissolve several drugs simultaneously with the same high drug loading capacity due to excellent solubility and outstanding formulation stability.34 In the case of the anti-cancer drug, paclitaxel (PTX), the solubility increase is approximately 50,000 fold. No additional formulation stabilizers or cryoprotectants are required. The weight ratio of the POx to the solubilized drug is close to 1:1.
Gene Delivery
POx have been studied in some detail as a platform for complexation of DNA. For example, Hsiue and co-workers used the well-known hydrolysis of POx to PEI to prepare block copolymers of PEtOx and linear PEI.35,36 The cationic PEI is well-known to condense DNA and help transfection of cells. However, PEI is also well-known for its significant cytotoxicity. A slightly different approach is the use of cationic POx, which can be prepared from monomers with amine-bearing side chains. These amines must be protected during polymerization or loss of control over the polymerization will occur.37 Schubert, et al. recently utilized this approach to prepare cationic POx based hydrogels for reversible DNA binding.38
POx-based Hydrogels
POx have been used to prepare hydrogels for many years.39,40 Also stimuli-responsive hydrogels of POx are well-known.41,42 Considering the low protein binding35 and the excellent synthetic versatility of POx, it is not surprising that POx-based hydrogels have been studied for drug delivery and for tissue engineering applications.43
Conclusions and Future Outlook
Polyoxazolines are synthesized via a controlled living cationic ringopening polymerization. This enables a variety of architectures including linear copolymers, star-shaped or branched structures, and bottle brushes. In addition to structural control, the different substituents on oxazoline-based monomers enable tunability for several properties, including not only functional groups, but also stimuli responsive behavior. POx has been shown to be biocompatible and is being investigated as an equal or superior alternative to polyethylene glycol. It has been used to form micelles in drug delivery applications as well as to form POx-drug and POx-protein conjugates. The current research trends indicate that applications using POx material will continue to expand throughout the drug delivery and tissue engineering fields.
References
Para continuar lendo, faça login ou crie uma conta.
Ainda não tem uma conta?