Synthetic Carbon Adsorbents for Host Cell Protein Removal in Monoclonal Antibody Purification
Introduction
Monoclonal antibodies (mAbs) are a class of critical biotherapeutic drugs. The demand for mAbs is steadily increasing. But the depletion of host cell proteins (HCP) in the manufacturing of mAbs remains a challenge. HCPs can represent an immune response risk after the administration of mAbs if not reduced to appropriate levels. Removal of HCPs is typically done via a Protein A (PA) chromatography capture step, carried out in bind- and-elute mode. Following the Protein A purification step, the feed is passed through two polishing steps in the form of ion exchangers (IEX), namely cation exchange chromatography (CEX) and anion exchange chromatography (AEX). The remaining impurities are mostly low molecular weight, hydrophobic HCPs, that are difficult to remove either because of their physiochemical properties1, or their non-specific association with the antibody2. These impurities represent the group of critical HCPs that need to be addressed in order to achieve the highest possible purification.
Efforts to further reduce HCP content beyond that achieved with the standard PA-IEX downstream processes are being considered One such solution is to add additional polishing steps like hydrophobic interaction chromatography (HIC) between the PA and IEX chromatographic steps. In this work, a post-Protein A (PPA) pool was applied to three different carbon-based adsorbents in flow-through mode to test their HCP depletion behavior under dynamic conditions.
Methods and Materials
Post Protein A Pool
The PPA pool was produced by treating a clarified cell culture harvest of CHO-K1 containing mAb02 with Protein A chromatography. For this purpose, a 205 x 26 mm (108 mL) column packed with ProSep® Ultra Plus Protein A resin was used on an ÄktaTM Pure 25 system. A high salt wash is typically performed to further reduce the HCP content; however, the salt wash was omitted here to keep the HCP content high for the purpose of challenging the adsorbents. The HCP content of the PPA used in this study was 6,000 ppm. After thirty minutes of virus inactivation, the PPA pool was titrated to pH 5 with 2 M tris(hydroxymethyl)aminomethane.
Adsorbent Materials
Two Carboxen® synthetic carbon adsorbents and a commercially available depth filter were investigated for their HCP removal capability and mAb recovery. Carboxen® 1032 and Carboxen® 569 are commercially available (Merck KGaA, Darmstadt, Germany) synthetic carbon adsorbents with particle sizes in the range of 20-40 mesh. For this study, customized 50 μm (approx. 270 mesh) particles of these two synthetic carbons were used. The depth filter was selected for comparative purposes. The depth filter adsorbent bed comprises of traditional naturally sourced activated carbon and cellulose.
An image of Carboxen® synthetic carbon particles is given in Figure 1. The synthetic carbons are highly engineered derivatized resins which have been designed to withstand high pressures of up to 110 MPa. Resins used in bio chromatography undergo plastic deformation and traditional activated carbons are known to fracture and generate fines at far lower pressures.
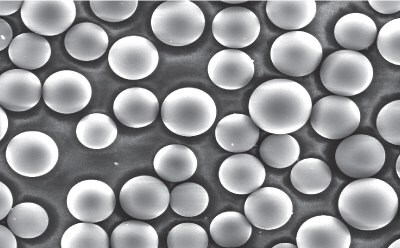
Figure 1.Carboxen® synthetic carbon particle.
Functionalization of carbon surfaces with oxygen functional groups alters the materials’ pH and hydrophobic/hydrophilic properties. The pH of Carboxen® synthetic carbons can be tailored from 2.5 to 10.5. The pH can be selected to meet the application’s needs. The surface chemistry dictates the hydrophobic/ hydrophilic properties and with proper oxygen functionalization, the synthetic carbon adsorbent can be made anywhere from hydrophilic to extremely hydrophobic. The Carboxen® synthetic carbons are activated through physical means. Therefore, there is no chemical residue left on the carbon that could create a chemical leaching concern in use. The carbon particles are of high purity and pass all the extractable and leachable tests as outlined in both USP 43 - NF 38 and Ph. Eur 10. As they are produced from a synthetic source, Carboxen® synthetic carbons are inherently free of heavy metals and other inorganic content found in naturally derived adsorbents.
While eight Carboxen® synthetic carbons are commercially available, 1032 and 569 were particularly selected since their properties are markedly different and thus a performance difference was anticipated between the two. Carboxen® 569 is highly hydrophobic and does not contain any ligand or attached surface functionality, and it can be thought of as a super phenyl phase. Carboxen® 569 has a point of zero charge at pH 8.8. Carboxen® 1032 is functionalized with oxygenated surface functional groups that give the material more of a hydrophilic character and a point of zero charge at pH 4.1. Material properties of the eight commercially available materials are given in Table 1.
Experimental Procedure
The Carboxen® materials were packed into Super Compact Columns (Götec Labortechnik GmbH, Germany) with a column volume of 300 μL. The columns’ inner diameter was 5 mm and the bed depth, 1.5 cm. The depth filter was used directly. Prior to the purification process, the depth filter was flushed with Milli-Q® water for 100 L/m² and conditioned with 50 mM acetate buffer for 300 L/m² at a flux of 300 L/h/m², corresponding to 2.5 mL/min. Packed columns containing Carboxen® synthetic carbons were flushed and conditioned with 50 mM acetate buffer for 50 column volumes at a flow rate of 0.5 mL/min.
For the HCP removal analysis, a pre-defined volume of 200 mL of the PPA pool, pH 5, was applied to each adsorbent. To maintain a residence time of 90 s within the devices, flow rates of 0.2 mL/min and 2 mL/min (corresponding to a flux of 240 L/h/m²) for the columns and the filter were applied, respectively. The PPA pool and each flow-through fraction were characterized by size exclusion chromatography (SEC) to determine the mAb load (onto each adsorbent) and mAb recovery. A Tosoh TSKgel® Super SW3000 SEC column was used on an UltimateTM 3000 UHPLC system with diode-array detector. HCP breakthrough was measured with ELISA. A CHO Host Cell Proteins 3rd generation ELISA-Assay Kit F550-1 from Cygnus Technologies was utilized. The procedure was carried out according to the manufacturer's instructions.
Results and Discussion
For comparison of the different adsorbents regarding their suitability for HCP depletion, the filter area was converted to volume based on its given dimensions. The HCP breakthrough was then plotted against the load of HCP (Figure 2A) and the load of mAb (Figure 2B) per adsorbent volume. Since the comparison was made at an HCP breakthrough of 10%, only this range is displayed.
Carboxen® 1032 had the best performance among the three materials and showed a very good depletion of HCPs. At an HCP breakthrough of 10%, 2300 g of mAb per liter of adsorbent could be applied on Carboxen® 1032 and 14 g of HCP per liter of adsorbent could be depleted. The depth filter performed the worst among the three materials tested for HCP depletion, with a depletion of about 3 g of HCP per liter of adsorbent after an application of 500 g of mAb per liter of adsorbent. Carboxen® 569 showed a performance in-between that of the other two materials tested. On Carboxen® 569, 900 g of mAb per liter of adsorbent could be applied, and 5 g of HCP per liter of adsorbent could be depleted. All three materials tested had a high mAb recovery, upward of 95%.
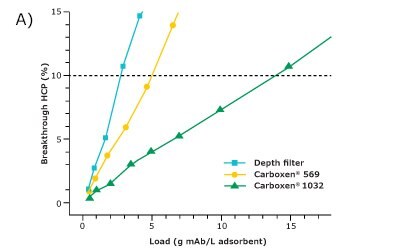
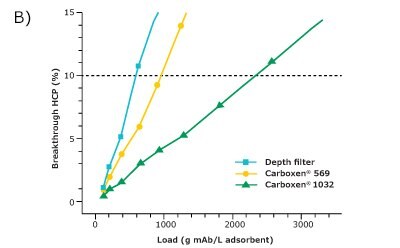
Figure 2. HCP breakthrough plotted against A) load of HCP and B) load of mAb per unit volume of adsorbent.
Carboxen® 1032 is assumed to be slightly negatively charged at pH 5, as its point of zero charge is lower than pH 5. Considering the excellent HCP retention obtained by this material, it is likely that most of the proteins are positively charged or uncharged under the given conditions. This observation leads to the assumption that most proteins have an isoelectric point of 5 or higher. In contrast, Carboxen® 569 is assumed to be strongly positively charged at pH 5 as it has a point of zero charge greater than pH 5. The depth filter contains activated carbon as an adsorbent and is also likely to be positively charged at pH 5. Therefore, the positively charged proteins are repelled by these adsorbents.
Carboxen® 1032 worked best, out of all the materials investigated in this study, and under these specific conditions. However, PPA pools are highly variable as are the purification conditions (e.g. pH and conductivity) and the polishing media needs to fit accordingly. There are eight commercially available Carboxen® synthetic carbons that cover a wide range of material properties with dispersion pHs ranging from 2.5 to 11, and from extremely hydrophobic to hydrophilic materials. Likely there is a Carboxen® synthetic carbon or a mixture that can optimize HCP depletion while maintaining high mAb yield in most mAb purification processes. This feat could be achieved by finding the best suited pH and conductivity of the PPA. For example, Carboxen® 569 is a strongly hydrophobic material. An increase in conductivity is expected to improve the HCP removal, as protein charges can be shielded by the added salt. Furthermore, pH variations could lead to different HCP depletion due to their different isoelectric points.
Conclusion
The Carboxen® synthetic carbons demonstrated excellent host cell protein reduction and high mAb yield. Carboxen® 1032 had the best performance among the three materials tested and showed a very good depletion of host cell proteins. At an HCP breakthrough of 10%, 2300 grams of monoclonal antibody per liter of adsorbent could be applied on Carboxen® 1032 and 14 grams of HCP per liter of adsorbent could be depleted. With the addition of a Carboxen® synthetic carbon polishing step to the standard PA-IEX downstream processes, difficult-to-remove lower molecular weight and hydrophobic HCPs can be depleted. With an added synthetic carbon purification step, downstream ion exchangers would bear a lower burden because of the reduction in impurities, so their durability can be increased. Ideally, the implemented step can replace one of the downstream ion exchangers. These points would lead to a reduction in production costs. In an alternative configuration, the Carboxen® synthetic carbon can act as a final polishing step to target the critical HCPs that are not removed in the standard PA-IEX process. Carboxen® synthetic carbons can ultimately lead to the production of a safer mAb product for the patient.
Choosing the right adsorbent or combination of adsorbents can be a challenge. Let us help you in selecting the appropriate adsorbent based on your specific application, Contact the Carboxen® Specialty Carbon Adsorbents expert.
Learn more about synthetic carbon adsorbent with these on-demand webinars:
High Purity Synthetic Carbon Absorbents for Challenging Separations
References
Para seguir leyendo, inicie sesión o cree una cuenta.
¿No tiene una cuenta?