Progress for High Performance Tandem Organic Solar Cells
Lingxian Meng, Xiangjian Wan, Yongsheng Chen
Nankai University
Material Matters, 2020, 15.1
- Introduction
- State-of-the-Art Tandem Organic Solar Cells
- Efficiency Prediction for Organic Tandem Solar Cells
- Summary and Outlook
- Acknowledgments
Introduction
Organic solar cells (OSCs) are widely considered one of the most promising photovoltaic technologies because they are low-cost, lightweight, flexible, and compatible with large-areaprinting technologies.1-3 Substantial progress has been made in the development of OSCs in the past decade. In particular, power conversion efficiencies (PCEs) have reached over 16% for single-junction OSCs through innovative material design and device optimization.4-6 However, despite the successes achieved by single-junction OSCs, their performance still lags far behind that of other technologies that are based mainly on inorganic materials, and their further improvement is subject to various limitations.1,3 First, the intrinsic, low-charge mobilities of organic materials restrict the thickness of the active layer and hinder sufficient absorption, which is detrimental for obtaining high photocurrent. Second, although the development of novel, narrow-band gap polymers and small molecule materials has widened the absorption spectrum range of the active layer of OSCs, the inherent narrow absorption windows of organic semiconductors still limits the photocurrent.3,7 In addition, singlejunction OSCs inevitably suffer from the serious thermalization loss of photon energy.8
One effective way to address these issues is to develop tandem devices with two or more sub-cells and complementary absorption spectra connected together. This approach can not only overcome the fundamental limits already outlined, more importantly, it makes full use of the advantages of organic materials, their diversity, easily tunable band structure, and facile synthesis.1,3 Furthermore, tandem devices maximize the absorption of OSCs and simultaneously reduce thermalization losses, significantly improving the PCEs of the devices.8-9
The most effective and widely studied tandem devices are the series-connected double-junction type, in which active layers are stacked in series through interconnection layers (ICLs).8 The front sub-cell based on wide bandgap materials absorbs light of shorter wavelength, and the rear sub-cell based on narrow bandgap materials make use of longer wavelength light. The complementary absorption of the two sub-cells maximizes the use of sunlight and further improves the performance of the device.8 Besides absorption spectra, there are many other factors that need to be considered in order to pursue higher PCEs for the series-connected tandem devices. First, on the basis of complementary absorptions, the two sub-cells should also possess well-balanced and high, short-circuit currents (Jscs) since the Jsc of tandem solar cells is limited by the sub-cells with smaller Jsc. Second, in series-connected tandem solar cells, the open-circuit voltage (Voc) is expected to be the sum of the sub- Vocs. The two sub-cells should have minimal energy loss to retain higher sub-Vocs. Third, the ICLs should be highly transparent and robust enough to protect underlying layers, and they should form ohmic contact with subcell active layers to ensure efficient charge extractions.3,8
Many explorations have been made for organic tandem cells in the past decade, and photovoltaic performance has recently reached 17%.10 In this review, the recent progress of tandem OSCs, especially that of non-fullerene (NF) systems, will be discussed. Subsequently, the efficiency prediction for tandem OSCs will be discussed through a semi-empirical analysis model reported earlier, which could provide guidance for material selection in each sub-cell to achieve high efficiency for tandem cells. Last, a general summary and outlook will be discussed at the end of the review.
State-of-the-art tandem organic solar cells
Most of the tandem OSCs reported in earlier literature are based on polymer/small-molecule donor materials and fullerene acceptors, in which PCEs of 11.6% and 12.7% have been achieved for polymer donors and small molecule-based tandem devices, respectively.11-12 Devices based on fullerene derivatives are expected to obtain a well-balanced Jsc through the appropriate selection of donor materials with different band gaps, since fullerene derivatives contribute little to the absorption of the blend films. However, the intrinsic narrow absorption bands of organic semiconductors do not cover a broad-enough range of the solar spectrum and thus lead to a low and unbalanced photocurrent.13 Furthermore, fullerene derivative-based, single-junction OSCs generally have high voltage loss, which limits the Voc of tandem devices.1,3 Recently, organic solar cells based on non-fullerene acceptors have made substantial progress and attracted significant attention. Compared with fullerene derivatives, non-fullerene acceptor materials, show many advantages, including 1) more efficient structure tunability (and thus higher bandgap adjustability) making it easier for these materials to complement the absorption of the donors and obtain a higher photocurrent, especially in the near-infrared region; 2) lower voltage loss, which allows a higher Voc in the tandem devices.1-3
Among various non-fullerene acceptors, molecules with Acceptor-Donor-Acceptor (A-D-A) architecture have achieved significant success. To date, most high efficiency OPV devices using non-fullerene acceptors with PCE over 10% are fabricated using A-D-A acceptors.1-3, 14, 15 In fact, A-D-A acceptors have been developed from donor molecules with A-D-A structures.
As shown in Figure 1, A-D-A molecules including donor and acceptor molecules have a symmetric structure with two electron withdrawing units on the outside. This unique chemical structure is expected to contribute to significantly improved photovoltaic performance of A-D-A molecules compared to other types of molecules, such as those with D-A-D structures. This is because the interaction between donor and acceptor materials in the bulk heterojunction (BHJ) devices plays an important role in the photovoltaic process, where the interaction mainly depends on the lowest unoccupied molecular orbital (LUMO) of the donor and acceptor after photo-induced excitation and generation of exciton. Proper interaction between two such LUMO orbitals is expected to favor an efficient charge separation. The outside LUMOs with all inside highest occupied molecular orbital (HOMO) for A-D-A molecules facilitate the exciton dissociation and charge separation process because of the easily formed exciton dissociation coupling with LUMOs of other donors or acceptors. This is one of the reasons that A-D-A molecules show excellent performance compared to other types of molecules. With these advantages, the tandem OSCs with A-D-A acceptor molecules have received much interest and achieved great progress in recent years.

Figure 1. Schematic of A-D-A structure.
In 2016, Chen et al. reported a tandem solar cell with a high Voc of 1.97 V. As shown in Figure 2, two acceptors, SF(DPPB)4 and IEIC were used in the front and rear sub-cells, respectively. Meanwhile, MoO3/ultrathin Ag/PFN was used as a dual-functional ICL. Finally, the tandem device achieved a PCE of 8.48%, which is limited by the low Jsc due to the much overlapped absorptions of the two subcells. With the high Voc, the above tandem device has been used for solar-cell-driven water splitting tests, demonstrating the great potential of high-voltage tandem cells.16 In 2017, Yan et al. reported a homo-tandem OSC based on an all-solution processed recombination layer with a mild, thermal-annealing treatment. They used the residue moisture in PEDOT:PSS (900181, 655201, 739332), to facilitate the hydrolysis of diethyl zinc precursor and then obtained the PEDOT:PSS/ZnO recombination layer. The mutually beneficial interaction enables the recombination layer to work efficiently with a mild thermal annealing. With this, the tandem device showed a PCE of 10.8% with a high Voc over 2.1 V.17

Figure 2.The tandem devices and chemical structures of the typical NF tandem solar cells.10,16-7,19-23
The innovation of efficient and low bandgap A-D-A type small molecule acceptors has created a great opportunity to fabricate higher efficiency tandem devices.3 The near-infrared absorption of these low band gap materials help to increase the Jsc of the tandem devices. In 2017, Alex et al. designed and synthesized a low-band gap electron acceptor, 4TIC, with absorption edge to ~900 nm. The single junction device with PTB7-Th (794333) as the donor material gave a PCE of 10.43% with Jsc of 18.75 mA/cm2, a fill factor (FF) of 0.72 and Voc of 0.78V. The high efficiency together with its small voltage loss makes PTB7-Th:4TIC a suitable rear sub-cell for tandem solar cells. The authors fabricated a series of connection tandem solar cells incorporating this rear cell and PBDB-T:ITIC (901099, 900799) as the front cell, and obtained an exceptional device performance of 12.62%.18 In the same year, Hou et al. reported a tandem device with an outstanding PCE of 13.8% with a Voc of 1.79 V, Jsc of 12.0 mA cm-2 and FF of 0.641. They designed a new NF A-D-A acceptor ITCC-M (900947) by replacing the phenyl rings in the end groups with α-methylthiophene groups. When blended with PBDB-T as the donor material, ITCC-M based devices showed a high Voc over 1 V, which is suitable as the front cell. Then, they selected the near-infrared absorption material, IEICO, as the acceptor and the low band gap donor material PBDTTT-E-T as the donor for the rear cell. A bilayer structure of ZnO/PCP-Na was used as the ICL.19 In their subsequent work, using the same ICL and device structure, Hou et al. fabricated a tandem OSC with a high PCE of 14.9%, in which J52-2F:IT-M and PTB7- Th:IEICO-4F were used as the front and rear cell, respectively. The complementary absorption of the subcells contributed to the improved Jsc and enhanced PCE.20
Recently, Forrest et al. demonstrated a tandem solar cell using a near-infrared A-D-A NF acceptor in the rear cell (PCE10:BT-ClC) and an evaporated small molecule in the front cell (DTDCPB). With an antireflection coating on the back of the glass substrate, a PCE of 15% was obtained. The large area device (1 cm2) showed a PCE of over 11.5%.21 Yang et al. employed a ternary non-fullerene system in rear sub-cell (PTB7-Th/FOIC/F8IC) to balance the voltage-current trade-off in tandem OSCs. A PCE of 13.3% was achieved.22 Huang et al. fabricated a tandem device using an NFA TfIF-4FIC (with a core of fused non-acyclic unit and end capping groups of 2-(5,6-difluoro-3-oxo-2,3-dihydro- 1H-inden-1-ylidene)malononitrile) in the front cell and the low-bandgap acceptor IEICO-4F in the rear cell. After device optimization, a high PCE of 15% was obtained.23
Based on the series of A-D-A molecules we developed, researchers in our group started to study tandem devices five year ago. At first, we fabricated a series of tandem devices using A-D-A molecules as donors and fullerene derivatives as acceptors. An outstanding PCE of 12.7% was achieved in 2017, which was the best value for fullerene based devices at that time.12 In subsequent work, we designed two new A-D-A small molecule acceptors named F-M (906972) and NOBDT with an absorption range from 300 to 900 nm. Using PBDB-T:F-M as the front sub-cell and PTB7- Th:NOBDT as the rear sub-cell, a conventional tandem device was fabricated with an outstanding PCE of 14.11%.24 Later, using two subcells with the same donor PBDB-T and two acceptors F-M and NNBDT with complementary absorptions to balance the Voc and Jsc, we fabricated an efficient inverted tandem OSC with an optimized PCE of 14.52%, a high Voc of 1.82 V, a notable FF of 74.7% and a Jsc of 10.68 mA cm-2.25
While the development of NF tandem OSCs has led to much progress in PCE, the resulting Jscs were still unsatisfactory, limiting tandem device efficiency. As a result, broadening the absorption range of the NF materials became a focus for generating further improvement of the Jsc of tandem devices. With the guidance of semi-empirical analysis (discussed in detail in the following section), we developed and reported on a tandem device that uses two A-D-A acceptor based subcells with complementary absorptions. PBDB-T: F-M was selected as the front cell because of its high voltage and suitable absorption range from 300-750 nm. A low band gap A-D-A small molecule acceptor, O6T-4F (906379), was used in the rear cell since the rear cell PTB7-Th: O6T-4F:PC71BM has absorption up to 1050 nm and higher Jsc — approximately matching the absorptions with the front cell. After device optimization, a record PCE of 17.36% was achieved, which is the highest value for tandem devices to date.10 The aforementioned photovoltaic parameters of NF tandem devices are summarized in Table 1.
Efficiency prediction for organic tandem solar cells
Previous efficiency predictions of tandem OSCs are mostly based on the use of fullerene acceptors.8 Since NF tandem OSCs have yielded better results as previously mentioned, the predictions based on NF tandem devices would clearly benefit from re-evaluation using these improved factors. In 2018, we proposed a semi-empirical model to direct the selection of materials for each sub-cell with optimized tandem cell efficiency. With the guidance of this semi-empirical analysis, a remarkable new record PCE of 17.36% has been achieved.10 The following is the main content of this model:
The semi-empirical analysis was based on a 2-terminal monolithic tandem cell with two subcells in series.10 Based on the equation of PCE= Jsc× Voc × FF/Pin (Pin is the power density of the incident light), to achieve a best PCE under AM 1.5G, the Jsc, Voc and FF need to be maximized simultaneously. Thus, firstly, the sub-cells need to have ideal Jsc in the corresponding absorption range and maximized Voc (meaning minimized Eloss). Also, there is a trade-off between the maximized Jsc and Voc as the multiplication of these two factors decide the eventual PCEs.10 The two photovoltaic parameters Jsc and Voc of a 2-terminal monolithic tandem cell are obtained as follows:

where E(λ) is the spectral irradiance in AM 1.5G, λ1 and λ2 are the absorption onsets of the rear and the front cells, h is Planck’s constant, c is the speed of light and q is the elementary charge. For tandem OSCs, the predicted PCEs should be obtained when the rear cell’s absorption is up to a certain range with fixed EQE, Eloss and FF. Then, the requirements for the front cell materials can be selected based on the modelling results and equation (1).10
Based on above assumptions, with fixed Eloss and FF of each sub-cell, we obtained the PCEs versus EQE and λonset, rear cell. Meanwhile, with fixed EQE and FF, the graphs of PCEs versus Eloss and λonset, rear cell were obtained.10 As shown in Figure 3B, if the λonset, rear cell is up to 1100 nm with an average EQE of 75%, FF being 0.75 and a typical Eloss of 0.6 eV, a PCE of ~ 20% can be reached. Moreover, the Eloss seems to make a bigger impact on the overall PCEs as shown in Figure 3C and Figure 3D. As shown in Figure 3C, with the fixed FF of 0.75 and EQE of 75%, when Eloss equals to 0.5 eV, the PCEmax of ~22.6% was obtained when the λonset, rear cell = 1060–1125 nm ; when the Eloss is 0.6 eV, a 20.2% PCEmax was obtained for λonset, rear cell = 1030–1100 nm; however, when the Eloss is as large as 0.7 eV, the PCEmax of 17.8% was obtained at a large range for λonset, rear cell = 900–1100 nm. With the increase of λonset, rear cell, the PCEmax gradually decreases. Also, with the increase of Eloss, the PCEmax will be obtained in a blue-shifted λonset, rear cell. Based on these results, there are several general rules that can be derived to achieve highperformance tandem devices. That is, sub-cells should have high EQE response, small Eloss and high FF, and the absorption of them should be complementary and sufficiently broad.10
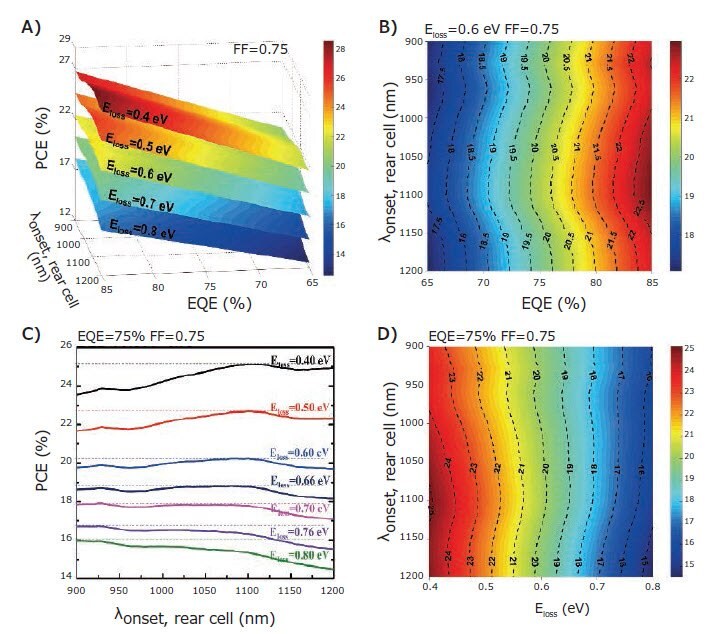
Figure 3. Predicted PCEs of 2T tandem solar cells based on semi-empirical analysis under AM 1.5G. A) PCEs versus EQE and λonset, rear cell with assuming the Eloss of each subcell in the range of 0.4–0.8 eV and a fixed FF of 0.75. B) PCEs versus EQE and λonset, rear cell with assumed Eloss of 0.6 eV and FF of 0.75. C) PCEs versus λonset, rear cell with Eloss of 0.4, 0.5, 0.6, 0.66, 0.7, 0.76 and 0.8 eV, FF of 0.75 and EQE of 75%. D) PCEs versus Eloss and λonset, rear cell with assumed EQE of 75% and FF of 0.75.10
Summary and outlook
In this review, we discussed the rapid development of nonfullerene acceptor tandem OSCs and methods for their further improvement. Herein, based on the results from the model analysis we have presented, one should pay particular attention to these specific rules to achieve higher PCEs: 1) The rear cell should have efficient absorption in longer wavelengths and the absorption edge should be in the range of 1050–1100 nm. 2) After the materials for the rear sub-cell are selected, the materials for the front cell should be selected based on the rule that the Jsc of each sub-cell must be the same for the optimal tandem cell. For example, if an Eg of ~1.18 eV (~1050 nm) for rear sub-cell is selected, the front cell material should have an Eg of ~ 1.72 eV (~ 720 nm) and a wide, efficient absorption in the range from 300 to 720 nm. 3) Careful attention should be paid to the Eloss. In addition to the rules 1) and 2) above, we recommend using materials with smaller Eloss to obtain higher Voc. 4) Prioritize the use of materials with higher FF, EQE, abd mobility.
Bu using these rules and the related modelling results, tandem solar cells with PCE of over 17% has been achieved.10 In the near future, PCEs of over 25% will be achieved provided that researchers can reach an EQE of 80%, Eloss of 0.45 eV and FF of 0.75 with a broad absorption onset of ~1050 nm using material design and device optimization. The realization of such results would hopefully push the commercialization of the organic photovoltaic technologies forward.
Acknowledgments
The authors gratefully acknowledge the financial support from MoST (2016YFA0200200, 2019YFA0705900), NSFC (51873089, 51773095, 21935007) of China, Tianjin city (17JCJQJC44500, 17JCZDJC31100) and 111 Project (B12015).
References
Para seguir leyendo, inicie sesión o cree una cuenta.
¿No tiene una cuenta?