Polymerase Chain Reaction
A Technical Guide to PCR Technologies
The polymerase chain reaction (PCR)1,2,3 has become one of the most widely used techniques in molecular biology. It is used in applications from basic research to high-throughput screening. While it is a powerful technique, the universal adoption and diverse range of applications is due to its apparent simplicity and relatively low cost. The technique is used to amplify specific, target DNA fragments from low quantities of source DNA or RNA (after a reverse transcription step to produce complementary DNA (cDNA), see Reverse Transcription). One major advantage of PCR is that target sequences can be amplified from a single copy of starting material, even when the template is degraded and contaminated with inhibitors. For example, studies have been performed on DNA extracted from ancient Egyptian mummies to identify the members of King Tutankhamun’s family4. PCR even features in major films and in most crime series television dramas at some stage in the “investigation,” even if somewhat over fictionalized.
PCR Cycling Process
A typical PCR consists of:
- Initial Denaturation: The reaction temperature is increased to 95 °C and the reaction is incubated for 2–5 min (up to 10 min depending on enzyme characteristics and template complexity) to ensure that all complex, double-stranded DNA (dsDNA) molecules are separated into single strands for amplification.
- Cycling:
- Denaturation: The reaction temperature is increased to 95 °C, which melts (disrupts the hydrogen bonds between complementary bases) all dsDNA into single-stranded DNA (ssDNA).
- Annealing: The temperature is lowered to approximately 5 °C below the melting temperature (Tm) of the primers (often 45–60 °C) to promote primer binding to the template.
- Extension: The temperature is increased to 72 °C, which is optimum for DNA polymerase activity to allow the hybridized primers to be extended.
- Denaturation: The reaction temperature is increased to 95 °C, which melts (disrupts the hydrogen bonds between complementary bases) all dsDNA into single-stranded DNA (ssDNA).
- Repeat: Steps 1–3 are performed in a cyclical manner, resulting in exponential amplification of the amplicon (Figures 2.1 and 2.2).
PCR consists of cycles of reaction heating and cooling. Each temperature plateau is used to control a defined stage of the reaction and the incubation times are dependent on the instrument, reaction plates or tubes and reagents. These should be optimized for each experiment, especially if increased detection sensitivity is required5.
The initial denaturation phase consists of a period at high temperature, during which the secondary structure of the complex double-stranded DNA (dsDNA) is melted to become single-stranded DNA (ssDNA). Since DNA is being subjected to high temperature, this phase needs to be long enough to separate all strands so that they are available for priming but not so long that the DNA is damaged. Degradation during the initial (or subsequent) denaturation steps or incomplete denaturation, results in decreased detection sensitivity5.
During the shorter denaturation step that initiates the cycling (10 sec to 1 min at 95 °C), the DNA strands of the target sequence separate to form single strands, just as in the initial denaturation stage. The reaction is then cooled to the primer annealing temperature.
The annealing step (30 sec to 1 min, at temperatures 45–60 °C), is required so that the primers bind to the complementary sequence on each of the DNA single strands. The primers are designed such that they bracket the target of interest and the region of sequence that lies between them is referred to as the amplicon. In general, the annealing temperature may be estimated to be 5 °C lower than the melting temperature of the primer-template DNA duplex.
The final stage is the extension step (20 sec to 1 min at 72 °C), which is performed so that the DNA polymerase extends the primer sequences from the 3’ of each primer to the end of the amplicon. A 1 min extension is typically sufficient to synthesize PCR fragments up to 2 kilobases (kb). To amplify larger fragments, the elongation step is extended at a rate of 1 min per kb. During the first extension, the template will not be length limiting and so templates will be synthesized that exceed the amplicon length. In subsequent extension steps, the amplicon length will be defined by the primer sequence at each end.
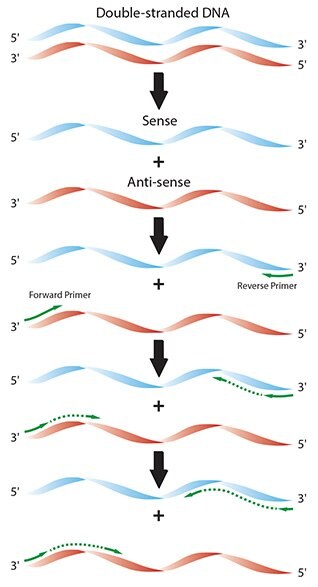
Figure 2.1.Diagram of the individual reaction processes in a typical PCR.

Figure 2.2.In a theoretical PCR, the quantity of target amplicon doubles with each cycle, leading to exponential amplification of the target sequence (X axis shows the PCR cycles, and the Y axis shows total number of amplicon molecules).
At the start of the second cycle, two forms of template are in the reaction; original DNA strands and the newly synthesized DNA strands, consisting of the primer sequence followed by variable lengths of the amplicon extended at the 3’ end. Remaining original DNA strands that were not primed in the first cycle may be captured in the second and result in molecules consisting of primer and extension product. The molecules from the first cycle that were primed and extended will be the template for primers that are complementary to the newly synthesized material.
In the third cycle, the newly synthesized target region DNA resulting from the second cycle comprises only the amplicon and therefore becomes the specific template.
Cycling is repeated continuously, resulting in exponential amplification of the copied sequences (Figure 2.2). The number of cycles required depends on the desired yield of PCR product. This, in turn, depends on the initial starting copy number and amplification efficiency. Since the relative concentration of the starting material to the end product is incredibly low (e.g., 1/5.511 if a single molecule is subjected to 40 cycles of amplification and 100% efficiency is achieved at each cycle, Figure 2.1), the resulting DNA at the end of the reaction is almost exclusively the PCR amplicon.
Although the theoretical PCR should result in continuous exponential amplification, the reaction eventually reaches a plateau phase. This appears to be due to nonspecific binding of the DNA polymerase to DNA products6,7. This is clearly visualized when using quantitative real-time PCR (qPCR) and monitoring changes in fluorescent labels (see Quantitative PCR).
At the end of the reaction, the amplification products are analyzed using gel electrophoresis. Depending on the quantity produced and the size of the amplified fragment, the reaction products can be visualized directly by staining the gel with ethidium bromide (Figure 2.3) or using more recently introduced dyes, such as BlueView™ 8.

Figure 2.3.An example of conventional PCR products resolved through an agarose gel stained with ethidium bromide. A duplex PCR was run to detect 2 targets simultaneously, each differing in size. Both fragments can be seen as distinct bands (image courtesy of Marion Grieβl).
PCR Assay Components
Below is a summary of the components that are required for PCR. Many of these are described in additional detail in dedicated chapters as well as in the detailed, standard PCR protocols at the end of this guide (Appendix A).
Template
There are several available methods for purification of RNA and DNA. While these are all suitable, they may not all be equal. Contamination of template with other materials from the sample source can result in PCR inhibition and in extreme cases, complete failure of the assay. There are some examples that demonstrate that contaminants may even result in reaction enhancement9,10. See Sample Purification and Quality Assessment for more details on template purification and quality control and Reverse Transcription for information concerning Reverse Transcription (RT) reactions. Typically, 10 ng to 1 μg of genomic DNA (gDNA) is added to 20–100 μL PCR assays, whereas cDNA synthesis reactions are diluted 1/5 to 1/10 and 5 μL is added to 20–50 μL reactions.
Primers
Oligonucleotide primers are typically 15–25 nucleotides in length with 40–60% GC and a Tm that is similar for each
member of the pair. A simple method to assess the Tm of short oligos (<25 bases) is to use the formula11:
Tm = 4(G + C) + 2(A + T)
See PCR/qPCR/dPCR Assay Design for more details on primer design and handling. The ideal starting temperature to use for annealing is estimated to be 5 °C less than the melting temperature. The annealing temperature can be optimized using a temperature gradient PCR block. A protocol for temperature optimization (using qPCR as an example) is given in Appendix A.
DNA Polymerase
There is a large variety of DNA polymerase enzymes and it is critical that the correct enzyme is selected by using the definition of the experiment (not simply the availability of a given enzyme in the lab freezer). For example, some enzymes are designed to be inactive at low temperatures and are used in hotstart protocols to reduce nonspecific amplification, while others have low error rates and are selected for amplification of fragments for cloning.
dNTPs
The concentration of each dNTP (dATP, dCTP, dGTP, dTTP) in the PCR is usually 200 μM. However, the dNTP concentration must be in excess and may need to be increased for amplification of long fragments or highly abundant targets. Recently, there have been enhancements to PCR buffer components, such as CleanAmp™ dNTPs12. These are modified nucleoside triphosphates that block DNA polymerase nucleotide incorporation. CleanAmp dNTPs are activated by the initial heating step and subsequent denaturing steps in typical hot start cycling conditions. This process limits the amount of available activated dNTPs during each cycle of PCR, which allows for more specific and efficient amplification of the desired product. In turn, they reduce, or completely avoid, mis-priming or primer dimer formation and therefore are an alternative to hot start DNA polymerases.
MgCl2 Concentration
Free Mg2+ ions are required as a co-factor for DNA polymerase activity; however Mg2+ ions form complexes with dNTPs, primers and DNA templates. For this reason, the optimal concentration of MgCl2 needs to be selected for each experiment by testing reactions containing different concentrations. MgCl2 concentration is typically between 1.5–5.5 mM but can be tested in the range 1–8 mM during optimization (see Assay Optimization and Validation).
Fluorescent Labels
Fluorescent dyes are incorporated into the reaction when the amplicon is to be detected directly, such as when using a qPCR or digital PCR (dPCR) set up. These are included in the buffer or attached to the primers or additional probes (see Quantitative PCR and Digital PCR Detection Methods).
Instruments
Originally, performing PCR was labor intensive because it was carried out by physically moving the reaction tubes between 3 water baths, each of which was set at the required temperature for denaturation, annealing and elongation. Oil was added to the top of the reaction to prevent evaporation and analysis was tricky to run without getting interference from the oil. Today, it is common to use one of the many specialized thermal cycling instruments that are commercially available. Plate and block formats vary between 48-, 96- or 384-wells and are compatible with multichannel pipettes. Most utilize a heated lid that negates the need for the oil overlay. However, there are high-throughput instruments based upon the original water bath principle13.
Application-specific PCR Modifications
Various derivations of PCR require reaction modifications. Some of these modifications for popular applications are included here.
Cloning
PCR may be used to amplify selected sequences for insertion into a vector. These sequences can be modified to include specific regions (tails) for cloning enzyme recognition. Primers directed to the vector are used to isolate fragments that have already been cloned into vectors. A low error rate DNA polymerase enzyme is necessary for the PCR steps.
Sequence Tag Sites
These are engineered into the 5’ end of the primers and are used during high-throughput sequencing when the specific tag is required as an indicator that a particular segment of a specific sample genome is present in a selected clone so that genomic sequence data can be deconvoluted and ascribed to the original source sample.
Site-directed Mutagenesis
To study protein function, a desired mutation may be introduced into a DNA sequence. The desired base change is engineered into the primers (towards the 5’ end), which also contain the required cloning enzyme restriction sites. An alternative approach adopts a multiple step protocol whereby primers that are specific to the target and contain the cloning sites are used in conjunction with primers containing the desired mutation.
References
Para seguir leyendo, inicie sesión o cree una cuenta.
¿No tiene una cuenta?