Methods of Synthesizing Monodisperse Colloidal Quantum Dots
Lutfan Sinatra, Jun Pan, Osman M. Bakr
Physical Science and Engineering Division, King Abdullah University of Science and Technology, Thuwal, Saudi Arabia
Material Matters, 2017, 12.1
Introduction
Colloidal quantum dots (CQDs) are semiconducting crystals of only a few nanometers (ca. 2–12 nm) coated with ligand/ surfactant molecules to help prevent agglomeration. As CQDs exist on a nanometer scale, they can offer unique properties via quantum confinement.1 It is possible to achieve highly tunable electrical and optical properties by adjusting the shape, size, and composition of a QD and in doing so, facilitate the use of QDs in a broad range of applications. QDs are particularly promising in optoelectronic devices such as light-emitting diodes, photovoltaics, photodetectors, lasers, and field-effect transistors.2–4
Since researchers first described the size-dependent properties of QDs at the beginning of the 1980s,5 a variety of different QD nanocrystals have emerged,6 and significant progress has been made in the realm of semiconductor particles, especially in terms of colloidal synthesis methods. The development of CQDs has been particularly promising in the area of solutionprocessable optoelectronic devices; for example, those involved in spin coating, ink-jet printing, blade coating, and screen printing have paved the way for the low-cost fabrication of largearea flexible devices.7
However, these developments have not been without their challenges. One particular challenge is in the synthesis of monodisperse and narrow size-distributed CQDs. Over the past 30 years, advances in the methods used to produce CQDs have resulted in the evolution of different approaches to synthesize them with uniform size. Monodisperse CQDs are significant because they possess relatively homogeneous properties that can be used in high-quality optoelectronic devices.
Herein we summarize a number of methods to synthesize CQDs, concentrating on the evolution of monodisperse cadmium- and lead-based CQDs—particularly CdSe, CdTe, PbS, and perovskite QDs—due to their wide-spread use and efficient properties. We discuss the various approaches for the batch synthesis of monodisperse QDs including hot-injection, heat-up, clusterassisted, and microwave-assisted synthesis methods. We also assess continuous flow-assisted synthesis as a means of producing CQDs on a large production scale and compare the merits of the batch and continuous flow-assisted approaches.
The Hot-Injection Method
The current approaches to synthesizing monodisperse CQDs are predominantly based on the work of LaMer and Dinegar, who described how the production of monodisperse colloids is dependent on a rapid nucleation followed by the controlled growth of the existing nuclei (Figure 1).8,9 Using this approach, Alivisatos and Bawendi et al. introduced the “hot-injection” method of synthesizing monodisperse CdS, CdSe, and CdTe QDs.10,11 To this day, the hot-injection approach remains the most common method by which monodisperse nanocrystals are synthesized. It involves the production of homogeneous nuclei via the rapid injection of organometallic reagents into a hot solvent. The reaction solution used during this process also contains surfactant molecules/ligands to prevent QDs from agglomerating. The ligands that are typically employed include alkylphosphine and alkylphosphine oxides (e.g., trioctylphosphine, Prod. No. 718165 and trioctylphosphine oxide, Prod. No. 223301), long-chain carboxylic acids (e.g., oleic acid, Prod. No. O1008), and long-chain amines (e.g., oleylamine).2 Once nucleation has occurred, a homogeneous diffusion-controlled growth is observed across the solution during which the larger QDs grow more slowly than the smaller QDs, resulting in a size-focusing effect. As the growth progresses, Ostwald ripening occurs, in which the larger QDs continue to grow while the smaller QDs dissolve due to their higher chemical potential.4 Until the point of saturation (Figure 1A), the average particle size increases and the concentration of the particle decreases. The hot-injection method is particularly effective because it offers a high level of control over the size of the particles and size distribution by allowing a rapid nucleation separated from the growth stage. By varying the temperature, concentration of the surfactants, and reaction time, it is possible to obtain QDs of various sizes. Additionally, this method has also proven to be effective at synthesizing various types of QD nanocrystals.

Figure 1. A) Schematic of the nucleation and growth stage for the preparation of monodisperse nanocrystals in the framework of the LaMer model. B) Representation of the simple synthetic apparatus employed to prepare monodisperse nanocrystals. Modified with permission from the Annual Review of Materials Science, Volume 30 Copyright Annual Reviews.
Recently, the hot-injection method has been effectively adapted to synthesize all-inorganic cesium lead halide perovskite (CsPbX3, X = Cl, Br, I) nanocrystals.12 CsPbX3 nanocrystals can be obtained by reacting Cs-oleate with a Pb(II)-halide in octadecene (Prod. No. 74740) at a high temperature (typically 140–200 °C) under N2 atmosphere. Prior to injecting the Cs-oleate, a mixture of oleylamine and oleic acid at a 1:1 ratio is added to the Pb(II)-halide solution in octadecene (at 120 °C and N2 atmosphere) to solubilize the Pb(II)-halide and stabilize the obtained nanocrystals. Due to the nature of the ionic metathesis reaction in CsPbX3 nanocrystals, the nucleation and growth kinetics are very rapid, with the growth taking place within just 1–3 seconds. Therefore, the size of the CsPbX3 nanocrystals can be tuned conveniently by varying the reaction temperature, but not varying the reaction time. The CsPbX3 nanocrystals produced via this approach exhibit bandgap energies tunable by size and composition. This method can be employed to create CsPbX3 nanocrystals that cover the entire visible spectral region with narrow emission line widths (12–42 nm) and exhibit a photoluminescence quantum yield (PLQY) ca. 50–90% (Figure 2).
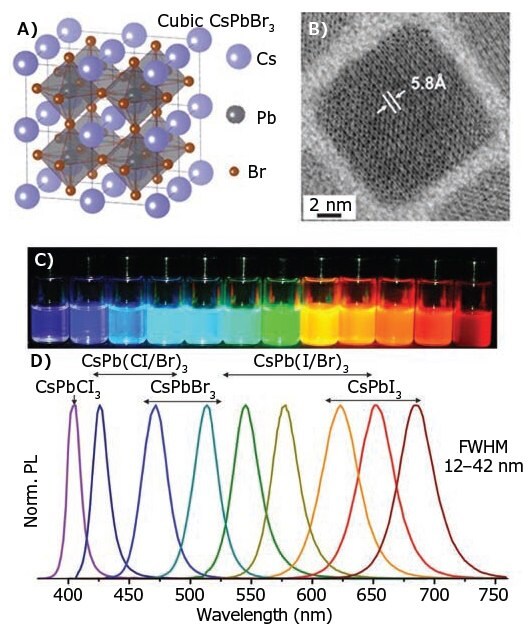
Figure 2. A) Schematic of the cubic perovskite lattice of CsPbX3. B) Typical transmission electron microscopy (TEM) images of CsPbBr3 NCs. C) Colloidal perovskite CsPbX3 NCs in toluene under UV light. D) Representative PL spectra. Reproduced with permission from Reference 12. Copyright 2015 American Chemical Society.
The Heat-Up Method
The heat-up method is a non-injection approach that involves steadily heating the precursors in the presence of ligand. The heat-up method is generally considered to be a more efficient approach to preparing nanocrystals because it allows them to be prepared in a single pot without an injection step. The majority of the heat-up synthesis methods results in a polydisperse particle size-distribution because nucleation events are spread over an appreciable period of time. Cao et al. found that it is possible to obtain monodisperse CQDs with this method if precursors with a suitable level of reactivity are used. In a similar strategy to the hot-injection approach, the key is to employ a homogeneous nucleation process through the use of precursors that are highly reactive only when they reach a certain temperature. In general, the precursors should exhibit no, or very low, reactivity below the desired temperature to allow the predominance of growth in the target nanocrystals (Figure 3).13
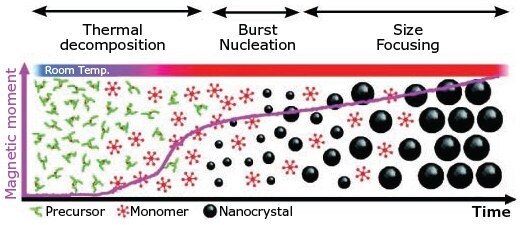
Figure 3. Schematic of the heat-up method.14
For example, it is possible to synthesize monodisperse CdSe QD nanocrystals in octadecene through the use of cadmium myristate, which decomposes at around 226 °C, and selenium powder (Prod. No. 229865), which decomposes at around 221 °C, as effective precursors at a growth temperature of 240 °C. In addition, selenium powder is only soluble in octadecene when the temperature exceeds 190 °C. As such, previous research has found that the nuclei appear when the temperature reaches around 210 °C, and this appearance is subsequently followed by constant growth. The concentration of the particles then increases to a maximum level before subsequently decreasing. This method proved to be an effective approach to synthesizing monodisperse (<5% standard deviation of sizes) and high-quality CQDs that have a PLQY of between 30–40%. In addition, a similar approach can be used to synthesize CdTe nanocrystals through the use of cadmium octadecylphosphonate and tributylphosphine telluride precursors.
The Cluster-Assisted Method
The cluster-assisted method utilizes very uniform clusters or seed particles within the reaction system. These act as nuclei and induce a controlled growth of the nanocrystals. The reaction is typically initiated by combining a known concentration of a small organometallic cluster with a coordinating solvent in the presence of semiconductor precursors (Figure 4A) before slowly increasing the temperature. This reaction seeding results in the clusters evolving into QD nucleation sites.

Figure 4. A) Schematic of cluster-seed method for synthesizing quantum dots. B) Graphic of concentration of the obtained quantum dots as a function of cluster concentration.15
Snee et al. reported the use of the cluster-seeded approach to synthesize CdSe QDs using (NMe4)4[Cd10Se4(SPh)16] and [Na(H2O)3]2[Cu4(SPh)6] clusters.15 They employed a typical synthesis process in which a certain quantity of the clusters was introduced into a mixture that consisted of octadecene (7 mL) and oleylamine (1 mL) with cadmium and selenium precursors (cadmium acetylacetonate, Prod. No. 517585 and diphenylphosphine selenide Prod. No. 802441 or tri-noctylphosphine selenide). The mixture was subsequently stirred for 24 hours at a temperature of 50 °C under N2 atmosphere. The study revealed that the presence of the cluster seeds directly impacted the concentration and size of the QDs. As Figure 4B shows, the resulting concentration of QDs was almost equal to the number of the cluster seeds (within 10%). Furthermore, the number of QDs produced via this method was approximately 20 times higher than that obtained via a standard rapid injection procedure that employs the same amount of precursors. In addition, increasing the cluster seed concentration decreased the size of the QDs due to the increasing number of QD nuclei competing for the finite number of available precursors. This approach has proven to be a versatile method of controlling the concentration of QDs and, furthermore, produces a large amount of QDs during a single reaction at a lower temperature.
The Microwave-Assisted Method
The microwave-assisted method of synthesizing nanocrystals offers excellent process control and allows elevated temperatures to be reached rapidly. This method is particularly convenient as the injection step is not required during the reaction, and the synthesis can take place under an air atmosphere. It is possible to avoid the variation in heating rate associated with conventional batch synthesis through the use of a microwave. When experimenting with the use of a microwave to synthesize nanocrystals, Strouse et al. were able to control the growth of CdTe and CdSe nanocrystals through selectively heating the chalcogenide precursor using microwave irradiation, which instantly activates the precursors that have a high polarizability. This activation is then followed by the nucleation and development of the nanocrystals.16 In the microwave-assisted method, the growth rate can be controlled via a combination of reactant concentration and microwave power, while the particle size can be controlled by adjusting the reaction temperature. This approach to synthesis has been found particularly versatile and reproducible, offering a reaction time below 3 minutes, a very small standard deviation (6% for CdSe and 12% for CdTe) in terms of size across ten different reactions, and emission line widths of about 27–28 nm (CdSe) and 40 nm (CdTe).
In a standard microwave-assisted synthesis, cadmium stearate in alkane solvent is combined with the selenium powder in tri-n-octylphosphine inside a microwave reaction vessel at a 1:5 molar ratio of cadmium to selenium. Hexadecylamine (Prod. No. 445312) is added to the mixture before a septum is used to seal the vessel. Subsequently, the vessel is placed in a microwave and heated to a temperature of 240 °C with a ramping period of 30 seconds at a power of 300 W and a holding period of 30 seconds at 240 °C. The reaction is cooled immediately to room temperature. A study by Kappe et al. compared the conventional hot-injection method with the microwave-assisted method and found that the quality of the QDs obtained was identical for both sets of experiments.17
The Continuous-Flow Method
The continuous-flow approach offers some distinct advantages over batch synthesis; in particular, it offers a more viable path to mass production. When using a continuous flow reaction method, it is possible to readily automate and control the reaction parameters, achieve a more efficient process of optimization, enhance the efficiency with which the precursors are mixed, and increase the scalability. Recent developments in the design of the reactor used in the continuous-flow approach have delivered significant improvements in terms of the automation of a large-scale chemical reaction. Two-phase segmented-flow approaches (gas–liquid or liquid–liquid) solve the problems of slow diffusive mixing of precursors and broad residence time distribution that are inherent in the single-phase laminar flow design (Figure 5A). A two-phase segmented-flow also offers enhanced mixing of precursors and optimum heat and mass transfer in smaller volumes (isolated droplets) of chemical reactions, which have been shown to result in more monodispersed nanomaterials that are of a higher quality.18,19
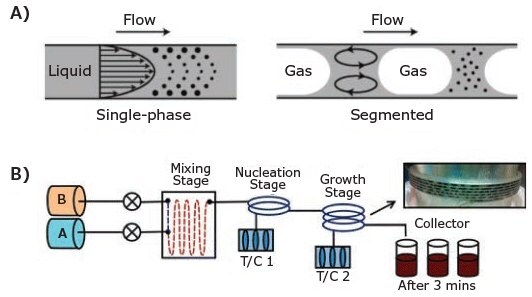
Figure 5. A) Schematic of mixing in single-phase and segmented gas–liquid flow synthesis. Reproduced with permission from Reference 18. Copyright 2005 Wiley Company. B) A dual-stage continuous-flow reactor setup that separates nucleation and growth stage.20
For example, PbS CQDs can be synthesized through the use of a dual-stage continuous-flow reactor system in combination with a two-phase segmented-flow approach (liquid–liquid). When the dual-stage system is employed, nucleation and growth take place in two separate reactors, allowing the application of different temperatures and subsequently providing more control over the nucleation process, as depicted in Figure 5B.20 Twophase segmentation is achieved by adding an inert, immiscible fluid (Fluorinert™ FC-70, Prod. No. F9880) into each precursor under stirring conditions. Precursor A contains lead oleate in octadecene, and precursor B contains bis(trimethylsilyl) sulfide (Prod. No. 283134) in octadecene. The two precursors are pumped into the mixer under N2 overpressure. They then progress to the nucleation reactor at the temperature of nucleation (TN) and, subsequently, the growth reactor at the temperature of growth (TG). The reaction can also be controlled by manipulating the total residence time (tR) of the reaction inside each reactor. By optimizing the parameters (TN, TG, tR) in the dual-stage system, high-quality PbS CQDs can be synthesized with quality similar to that produced via the hotinjection method but with a greater production scalability. The typical production yield is 2.4–2.5 g/h.
Conclusions
Thanks to advances in synthetic methods, it is possible to synthesize monodisperse CQDs using a variety of approaches. Many of these approaches attempt to achieve similar outcomes in that they employ comparable mechanisms to obtain the homogeneous nucleation and controlled growth of monodisperse CQD nanocrystals. The hot-injection method is currently the most popular synthetic strategy to synthesize monodisperse CQDs. However, other methods, such as heat up, cluster assisted, microwave assisted, and continuous flow can offer sufficient advantages and versatility in terms of process efficiency. The heat-up method generally allows nanocrystals to be prepared in a single pot without an injection step. The microwave-assisted method of synthesizing nanocrystals is similar to the heat-up method but allows elevated temperatures to be reached rapidly, completing synthesis within minutes. The cluster-assisted method provides control over the concentration of QDs and enables a large amount of production but requires the judicious design of a suitable seed cluster. The continuousflow assisted method represents an automated approach to producing high-quality CQD nanocrystals on a production scale. In the fabrication of QDs, a wide array of methods and synthetic tools are available. The selection criteria for each approach are dependent on the chemical composition and temperature requirements of the materials system, as well as the target production scale.
References
계속 읽으시려면 로그인하거나 계정을 생성하세요.
계정이 없으십니까?