Chemistry at Surfaces with Self-Assembled Monolayers and SAMDI-ToF Mass Spectrometry
Prof. Milan Mrksich
Department of Chemistry, The University of Chicago Chicago, IL
Material Matters Volume 3 Issue 3
Modern synthetic chemistry permits the construction of elaborate molecular structures and has been vital to the development of pharmaceuticals, catalysts, and functional polymers. Chemists no longer ask whether, but rather how efficiently, a target structure can be prepared. A vastly different expectation holds when these same reactions are applied to the elaboration of surfaces. While surfaces having well-defined structures and that present a broad range of functional groups are easily prepared, the difficulty in characterizing the products of interfacial reactions makes it difficult to carry out even the simplest transformations. Ironically, interfacial reactions are substantially easier to perform than corresponding homogeneous phase reactions— because the reaction workup requires only that the surface be rinsed of the reaction mixture; however, the characterization of products, yields and rates is enormously more difficult. For reactions performed in solution, the products are easily isolated, purified and characterized using NMR, IR and other spectroscopic methods. The small amount of product present when these same reactions are performed at a two-dimensional interface makes these analytical tools useless and instead requires the use of several methods that are sensitive but limited in the structural information they provide.1
This article describes the combination of self-assembled monolayers with matrix-assisted laser desorption-ionization mass spectrometry—a technique termed SAMDI MS—that permits a rapid characterization of products resulting from interfacial reactions.2 The self-assembled monolayers are an attractive platform for surface chemistry because they are easily prepared by immersing a gold-coated substrate in a solution of terminally-substituted alkanethiols under normal laboratory environments and because they are synthetically flexible. This latter property stems from the compatibility of the assembly process with alkanethiols carrying a wide range of terminal functional groups—since the chemisorption of thiol for gold is quite specific—and because the resulting monolayers are thermally stable and compatible with a wide range of solvents and reagents.3 The growing availability of alkanethiol reagents from commercial suppliers and the lack of a need for significant instrumentation and facilities make these substrates accessible to synthetic chemists. The development of the SAMDI MS technique finally provides a rapid and routine method of characterizing reactions of molecules attached to selfassembled monolayers and could prompt a significant growth of basic and applied research in molecular surface chemistry.
The first examples of characterizing self-assembled monolayers with laser desorption mass spectrometry came from the laboratories of Hemminger, Fritsch, Wilkins and Hanley.4-6 Fritsch and Wilkins, for example, used a 308 nm laser to desorb monolayers and were able to observe alkanesulfonates that result from oxidation of alkanethiolates. At the time of that work, the limited availability of commercial instruments for laser desorption mass spectrometry and the still limited number of examples of interfacial reactions on monolayers prevented a wider adoption of the method. Some years later, when challenged by the difficulties in characterizing interfacial reactions, we found that commercial instruments for matrixassisted laser desorption-ionization mass spectrometry could be applied to self-assembled monolayers that were functionalized with a broad range of molecular groups.7 In a typical experiment, a solution of the common matrix molecules used in SAMDI MS is applied to a monolayer and allowed to dry. Irradiation of the monolayer with a nitrogen laser results in efficient release of the alkanethiols—or the analogous disulfides—from the gold substrate and reveals the masses of these molecules (Figure 1). In a first example, we used this to characterize the cycloaddition of pentamethylcyclopentadiene to a monolayer-tethered maleimide group, the formation of an amide, and the deprotection of a tert-butyl ester. In each case, the SAMDI spectrum showed peaks that correspond to the substituted alkanethiols, or their disulfides, before and after the reaction. SAMDI complements other spectroscopic techniques in that it provides information on the total mass of the alkanethiols, rather than on the identities of functional groups present in the molecules. Further, the rapid acquisition of a SAMDI spectrum—the time to load and analyze a sample is less than ten minutes—makes this method useful for assessing reaction yields and confirming the presence of the anticipated product.
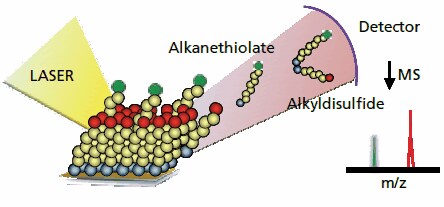
Figure 1.Self-assembled monolayers can be characterized using matrixassisted laser desorption-ionization mass spectrometry in a technique termed SAMDI MS. A nitrogen laser is used to desorb the monolayer to give alkanethiolate and the corresponding disulfide molecules. The mass spectrometer reports the mass-to-charge ratio for these molecules and can provide information on the products, yields and rates of interfacial reactions
Since this early report, SAMDI has been used in a broad range of applications, including the characterization of products resulting from electrochemical reactions of the monolayer,8 the combinatorial discovery of reactions,9 and many examples of enzyme-mediated transformations.10-13 Three examples, described below, demonstrate the unique capability that SAMDI offers in characterizing the products that result from interfacial reactions at self-assembled monolayers, and in turn enabling a broad range of studies that use functionalized surfaces.
The first example addresses the reactions of monolayers that present a terminal alkyne functional group. The basepromoted exchange of the terminal hydrogen atom with a deuterium atom represents a straightforward reaction yet one that is very challenging to characterize when performed on a monolayer. The SAMDI spectrum of a monolayer prepared from a mixture of an alkyne-terminated and a methyl-terminated monolayer revealed peaks corresponding to the mixed and the symmetrical disulfides. Treatment of the monolayer with sodium hydride and then deuterium oxide afforded exchange of the terminal hydrogen atom, as verified by the increase in mass of the disulfides by one and two Dalton, respectively (Figure 2). This example reveals both the straightforward application of mass spectrometry to characterizing an interfacial reaction and also the good mass resolution inherent to the method. This method was also used to demonstrate the hydration of the alkyne to give a methylketone and a Sonagashira coupling to give the phenylacetylene.
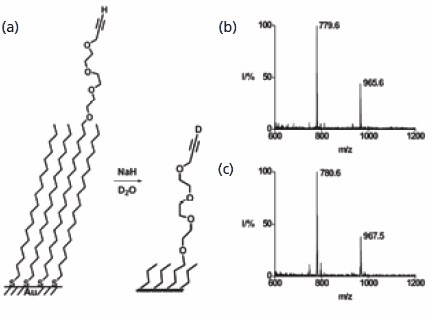
Figure 2.SAMDI MS is used to characterize the exchange of hydrogen for deuterium in a terminal alkyne. The mass spectrum prior to reaction shows a peak at m/z of 779.6 that shifts to 780.6 after the reaction. The corresponding disulfide shows the expected mass increase of two Daltons.
The second example addresses the development of multistep synthetic sequences for elaborating the structures of self-assembled monolayers. Monolayers are frequently used in ‘biochip’ applications, where surfaces that present an array of peptides, carbohydrates or small molecules are used to identify substrates for enzymes or ligands for proteins from a large set of possible interactions.14 In these applications, the molecules that are tethered to the monolayer are often first synthesized using established methods and then immobilized to a monolayer using appropriate conjugation chemistries. For certain classes of molecules—for example, oligosaccharides—the time and expense required to prepare the molecules limit the sizes of arrays that can be prepared.
Early work with peptide and oligonucleotide arrays took advantage of methods to directly synthesize the molecules on the substrate and thereby rapidly and efficiently assemble arrays of hundreds to thousands of biomolecules on the surface.15 These examples required a substantial effort to develop and optimize the sequences of interfacial reactions used in array synthesis, with the most significant challenges being in characterizing the products and yields of the interfacial reactions. We recently used SAMDI to develop routes to preparing arrays of oligosaccharides by performing multi-step syntheses directly on the monolayer.16
The strategy used to synthesize an array of disaccharides is shown in Figure 3 and begins with a monolayer that presents a phenol group at a density of 5% against a background of tri(ethylene glycol)-terminated alkanethiolates. The former serves as the nucleophile for attaching the carbohydrate building blocks and the latter are effective at preventing the non-specific adsorption of protein and therefore are important for subsequent biochemical assays of the immobilized saccharides.17 A monosaccharide that was triacetylated and had the fourth hydroxyl group protected as the levulinate ester—to permit a selective deprotection in a following step— was prepared as its trichloroacetimidate and activated with trimethylsilyl trifluoromethanesulfonate for coupling to the phenol group. The monolayer was then treated with hydrazine to remove the levulinate group and then treated with a second monosaccharide reagent to give the fully protected disaccharide. Treatment with sodium methoxide led to removal of the acetyl groups and gave the immobilized disaccharide. SAMDI spectra showed that each step proceeded in high yield and gave the expected products (Figure 3). This sequence was applied to the preparation of an array of twenty four distinct disaccharides—which could be accomplished in less than six hours—and then used to profile the substrate specificities of a glycosyltransferase enzyme. Again, the use of mass spectrometry allowed the products of the enzyme reaction to be identified directly.
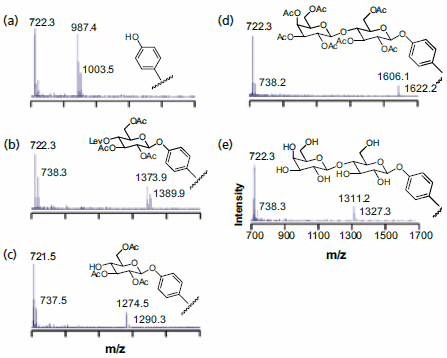
Figure 3.SAMDI MS was used to characterize the surface after each step of a disaccharide synthesis on a monolayer substrate: (a) monolayer presenting the phenol group; (b) coupling of the first carbohydrate; (c) selective removal of the levulinate protecting group; (d) coupling of the second carbohydrate; (e) final deprotection.
A third example addresses reactions of DNA oligonucleotides. DNA arrays that contain tens of thousands of distinct sequences are now commonly used to profile patterns of gene expression in cell cultures, and more recently have been used to identify protein-DNA binding interactions.18 The arrays use fluorescent labels to detect binding interactions and for this reason have not been applicable to studies of chemical reactivity including, for example, the covalent adducts formed on treatment of DNA with reactive small molecules. Our approach to use SAMDI to characterize the reactions of immobilized DNA begins with a monolayer that present a maleimide group at 5% density against a background of tri(ethylene glycol) groups.19 To attach the DNA, we first immobilized a biotin-terminated alkanethiol and then bound a streptavidin protein to give a surface that was used to capture a biotin-labeled oligonucleotide duplex. We treated an array of duplexes with the anti-cancer drug cis-platin and used SAMDI to identify the mono- and di-adducts formed in the reaction (Figure 4). The flexibility of the SAMDI method, together with the mature methods for preparing and applying DNA arrays may enable a significant extension of the applications that can be pursued with the arrays.
![Chemistry at Surfaces with Self-Assembled Monolayers and SAMDI-ToF Mass Spectrometry reaction of a biotinylated duplex DNA (5’-biotin-TTT TAT ATA CGT ATA TCG) with cis-[Pt(NH2)2Cl2]](/deepweb/assets/sigmaaldrich/marketing/global/images/technical-documents/articles/materials-science-and-engineering/polymer-synthesis/biotinylated-duplex.gif)
Figure 4.SAMDI MS was used to characterize the products resulting from reaction of a biotinylated duplex DNA (5’-biotin-TTT TAT ATA CGT ATA TCG) with cis-[Pt(NH2)2Cl2]. Spectra are shown for reaction times of 0, 4 and 21 hr (A, B and C, respectively).
The examples summarized in this review illustrate the value of SAMDI for rapidly assessing the products and yields of interfacial reactions at self-assembled monolayers. No methods, or combinations thereof, yet exist that can provide comprehensive information on the structures of molecules attached to surfaces, as do the techniques of NMR and x-ray diffraction for molecules prepared in larger quantities. Currently, studies in surface chemistry instead use several methods to assemble an understanding (often times incomplete) of interfacial structure, including infrared spectroscopy to identify functional groups, x-ray photoelectron spectroscopy to determine the elemental composition and ellipsometry to measure the thickness of a monolayer. By providing the molecular weight of the alkanethiols, SAMDI complements these methods and provides molecular information that is not available with other methods. Most significantly, this method is straightforward to use and provides the synthetic chemist with information that can be used to develop and implement a wide range of chemistries on monolayers. These benefits should enable the development of surfaces having complex structures for basic and applied studies in a broad range of disciplines.
References
To continue reading please sign in or create an account.
Don't Have An Account?