CytoSoft® Elastic Modulus Plates
An Innovative Tool to Analyze the Effect of Matrix Stiffness/Rigidity on Regulating Cellular Behavior
Mechanotransduction refers to the processes through which cells sense and respond to mechanical stimuli by converting them to biochemical signals that elicit specific cellular responses. Focal adhesions are large macromolecular assemblies through which mechanical force and regulatory signals are transmitted between the extracellular matrix (ECM) and an interacting cell. Cells in the body interact with their local 3D microenvironments which can vary based on tissue location and disease states. Cells reside in various matrix stiffnesses throughout the body. The mechanical property that measures the stiffness of a material is termed Young's elastic modulus and is expressed in kilopascals of pressure. Tissue culture plasticware has an elastic modulus of 1X107 kPa whereas tissues/organs have much lower values ranging from 0.2-64 kPa. In order to create more relevant in vitro cell models, researchers have started to grow on softer 3D hydrogels and substrates that more closely represent their native tissue rigidities. For example, changing to a stiffer substrate alters the differentiation potential of human mesenchymal stem cells to favor bone formation over cartilage and adipose tissues1.
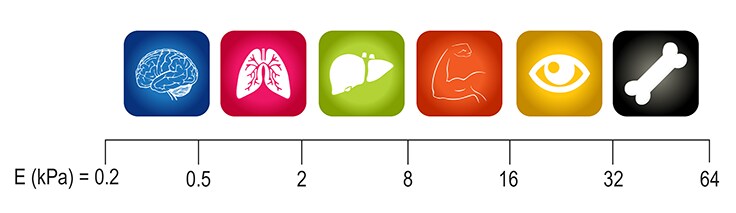
Figure 1. Native matrix stiffnesses of various in vivo tissues.
CytoSoft® elastic modulus plates are used to culture cells on substrates with various defined rigidities covering a broad physiological range (0.2kPA- 64kPa). On the bottom of each well, there is a thin layer of specially formulated biocompatible silicone, whose elastic modulus (rigidity) is carefully measured. The surfaces of the gels in CytoSoft® products are functionalized to form covalent bonds with amines on proteins. This chemical functionalization is stable and the reaction does not require a catalyst, facilitating the coating of the gel surfaces with matrix proteins and plating cells. For example, coating with an ECM protein, such as PureCol® (5006), is recommended before plating cells.
The silicone substrates of CytoSoft® plates are optically clear and have a low auto-florescence. The layer of silicone in each well is firmly bonded to the bottom of the well. Unlike hydrogels (such as polyacrylamide gels), silicone gels are not susceptible to hydrolysis, do not dry nor swell, are resilient and resistant to tearing or cracking, and their elastic moduli (rigidities) remain nearly unchanged during extended storage periods. CytoSoft® products accommodate the harvesting of cells using enzymes such as trypsin and collagenase. There is no biochemical breakdown of the substrate during or after enzyme treatment, and there are no residuals of the substrate in the sample retrieved from a CytoSoft® plate. For researchers who are unsure on which stiffness to use, we offer a Discovery Kit (5190) that has various elastic moduli of approximately 0.2, 0.5, 2, 8, 16, 32 and 64 kPa in 7 individual 6-well plates
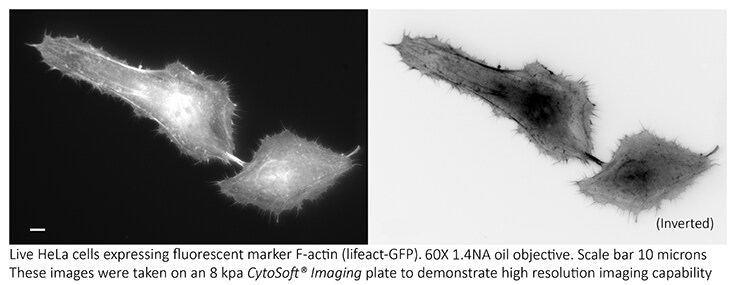
Figure 2. Fluorescent cell imaging of F-actin in HeLa cells using CytoSoft® imaging plates (8 kPA).Cell migration and adhesion can be analyzed by monitoring the expression of F-actin filamentous cytoskeleton protein.

Figure 3. Primary human dermal fibroblast matrix stiffness optimization.A elastic modulus of 8 kPA is an optimal matrix stiffness for dermal fibroblasts showing a reduction in F-actin stress fibers and increased cell adhesion (Vinculin) when compared with 0.2 or 64 kPa matrix stiffnesses.
Cytosoft® References
References
To continue reading please sign in or create an account.
Don't Have An Account?