3D and 4D Printing Technologies
Wonjin Jo, Kyung Sung Chu, Heon Ju Lee, Myoung-Woon Moon
3D Printing Group, Computational Science Research Center Korea Institute of Science and Technology, 02792, Seoul, Republic of Korea
Material Matters, 2016, 11.2
Developments in 3D Printing Technology
Three-dimensional (3D) printing technology, also called additive manufacturing (AM), has recently come into the spotlight because of its potential high-impact implementation in applications ranging from personal tools to aerospace equipment. Even though 3D printing technology has only recently emerged as a hot topic, its history can be traced back to 1983 when the first 3D printer was created by Charles W. Hull, co-founder of 3D Systems.
Widened Access to 3D Printers
Since then, new and wide-ranging applications and markets for 3D printers have appeared rapidly, especially with the expiration of a number of core 3D printing patents owned by Stratasys Inc. and 3D Systems Inc. Users can easily build or modify 3D printers by themselves or take advantage of the rapidly growing availability of inexpensive 3D printers. The recent availability of highly capable 3D design software and 3D design websites (e.g., Shapeway and Thingiverse) allows the sharing of user-created free 3D digital design files or models, leading to more access to 3D printers and additional proliferation of 3D printing technology. When compared to traditional manufacturing technologies such as casting, machining, and drilling, 3D printing is considered an efficient technology in the areas of energy and materials, utilizing up to 90% of materials and providing up to 50% energy savings.1
As 3D printing becomes more than just a simple production process, it has come to support a convergence of technologies and applications such as sports equipment, food packaging, and jewelry, as well as products in the high tech fields of aerospace, medicine, architecture, education,2,3 automotive industry, military support, and others.
Popular Applications
Fashion: At the 2016 New York Fashion Week, two unique 3D printed dresses were unveiled. These masterpieces were produced through a collaboration between fashion designers and the 3D printing company, Stratasys.4 The complex designs (e.g., mixing a variety of interlocking weaves, biomimicking natural animal textures) and cutting-edge material (e.g., nano-enhanced elastomeric 3D printing material) gave the dresses durability and flexibility.
Regenerative medicine: The area of regenerative medicine has also achieved impressive applications within the 3D printing field. Dr. Anthony Atala’s team from the Wake Forest Institute for Regenerative Medicine has successfully used 3D printing technology to fabricate living organs and tissue (including muscle structures, and bone and ear tissue).5,6 These bioprinted body parts are capable of generating functional replacement tissue.7
Aerospace: NASA has also been implementing 3D printing techniques and 3D printers to develop materials that allow astronauts to repair or replace essential parts and build structures in space. NASA recently collaborated with researchers at Washington State University to fabricate a replica of a moon rock using raw lunar regolith simulant and 3D laser printing technology.8,9
Construction: The assembly of modular construction materials using giant 3D printers for use in the housing industry has gained significant interest, especially for poorer countries, during natural disasters, or sudden emergencies. Some 3D companies have succeeded in building houses or bridges with cement, sand, or concrete materials.10–12
4D Printing
The rapidly decreasing cost, improved software design, and increasing range of printable materials have helped to bring about a new technology called four-dimensional (4D) printing. 4D printing provides printed objects with the ability to change form or function with time according to various stimuli such as heat, water, current, or light (Figure 1A).13 The essential difference between 4D printing and 3D printing is the addition of smart design, or responsive materials that cause time-dependent deformations of objects.
This review covers both 3D and 4D printing processes and shows the materials related to different printing types.

Figure 1. A) Schematic of 1-, 2-, 3-, and 4D concepts. B) The process of 3D and 4D printing technology involves three general stages: (1–2) modeling; (3–4) printing; and (5) finishing.
The Process of 3D and 4D Printing Technology
3D printing is the process of fabricating objects by building up materials layer by layer. Figure 1B shows the 3D printing process from modeling to final printing. Based on the use of computer-aided design (CAD) describing the geometry and the size of the objects to be printed, a complicated 3D model is created in a printable standard tessellation language (STL) file format (Figure 1B1, 1B2). Then, it is sliced into a series of digital cross-sectional layers in accordance with the layer thickness setting (Figure 1B3). Upon completion of the model, the object is fabricated by a 3D printer through the layer-by-layer fabrication process based on a series of 2D layers to create a static 3D object (Figure 1B4, 1B5). 3D printing can involve different types of materials such as thermoplastic polymer, powder, metal, UV curable resin, etc.
Four-dimensional printing incorporates a time component to the 3D printed objects, making the design process more important. 4D-printed structures must be preprogrammed in detail based on the transforming mechanism of controllable smart materials that incorporate timedependent material deformations.13 Figure 2A–C show 3D structures that self-fold based on the thermal activation of spatially variable patterns printed with a variety of shape memory polymers. Each polymer has a different thermal-dependent behavior that can make the box self-fold in a time-sequential manner based on smart design and thermomechanical mechanisms.14 The choice of materials for 4D printing is significant, however, because most 3D printing materials are designed only to produce rigid, static objects. Recently, some smart shape alloy/polymer memory materials have been developed to utilize their self-assembled behaviors driven by heat, UV, or water absorption-driven as shown in Figure 2D–F.13,15 For example, the temperature-responsive artificial hand shown in Figure 2F was printed with a temperature-responsive TPU (thermal polyurethane) filament. It has the ability to contract or expand in response to specific temperatures. In addition, multi-materials having different environmental behaviors are also useful in 4D printing. A research group at the Massachusetts Institute of Technology used two different materials with different porosities and water-absorption abilities to print transformable structures.16,17 It was composed of a porous water-absorbing material on one side and a rigid waterproof material on the opposite side. When exposed to water, the water-absorbing side increased in volume while the other side remained unchanged, resulting in shape deformation.
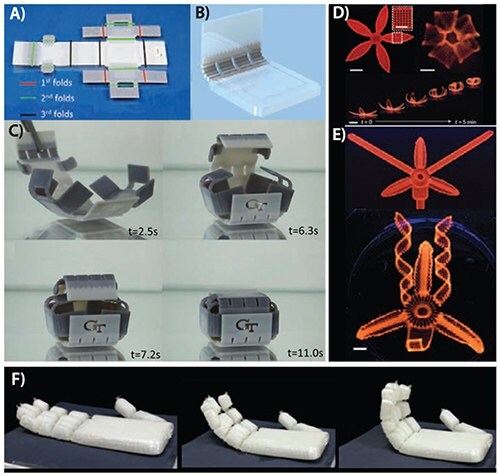
Figure 2.A–B) The design of the folding box with different materials assigned at different hinges. C) Upon heating, the programmed 3D printed sheet folds into a box with a self-locking mechanism. Copyright 2015, rights managed by Nature Publishing Group. D–E) The resulting swollen flower structures were generated by biomimetic 4D printing with composite hydrogel and cellulose fibrils. Copyright 2016, rights managed by Nature Publishing Group. F) The temperature-responsive artificial hand was made with temperature-responsive TPU filament.
Classification of 3D and 4D Printing Technologies
The 3D and 4D printing technologies are classified into different printing processes, defined mainly by the types of materials used. The selection of materials has a direct influence on the mechanical or thermal properties, as well as the transformation stimuli of the finished objects. This section describes the three most common types of 3D and 4D printing and reviews the most frequently used materials for these processes.
Fused-deposition Modeling (FDM)
The FDM method operates by extruding thermoplastic materials and placing the semi-molten materials onto a stage to fabricate a 3D structure layer by layer.18 More specifically, the thermoplastic filament is first led to an extruder which feeds and retracts the filament in precise amounts. The filament is melted by a heater block set to the melting temperature and moved through the extrusion nozzle tip by two rollers. The extruded filament is deposited as the print head traces the design of each defined cross-sectional layer of the desired structure by a digitally positioned mechanism. Then, the stage moves to the Z position in accordance with the setting value of layer thickness. These steps are repeated to complete fabrication of the 3D structure.
One advantage of FDM is the availability of a variety of filament materials as shown in Figure 3. A wide selection of FDM filaments are commercially available with different strength and temperature properties, such as ABS (acrylonitrile butadiene styrene, Product No. 3DXABS001–3DXABS0016), nylon ( Product No. 3DXION001–3DXION004), PET (polyethylene terephthalate, Product No. 900095 and 900125), TPU (thermal polyurethane, Product No. 900126 and 900128), POM (polyoxymethylene), PC (polycarbonate), HIPS (high impact polystyrene), and PVA (polyvinyl alcohol), among others. In addition, some materials can be used as a raw material for mixing with other functional materials to improve specific functions. Among them, the PLA (polylactic acid) filament is a popular choice due to the many available properties as shown in Figure 3. Due to the thermoplastic behavior, many FDM filaments can also be used as 4D materials under applied heat change.

Figure 3.Thermoplastic filaments for Fused-deposition Modeling (FDM). The FDM-printed flower was made with a color changed filament under UV exposure.
Powder Bed and Inkjet Head 3D Printing (PBP)
The PBP process is an adaptation of inkjet printing. In this process, a layer of powder is first deposited and rolled to ensure uniform thickness, then the inkjet print head drops binder in a specified pattern as it moves, to form a single layer of a printed object across the bed of powder. The next powder layer is distributed over the deposited liquid binder, and this process is repeated, with each layer adhering to the last. Support structures are not required in PBP due to the ease of removing unbound powder using an air gun, after solidification of the finished object. The use of multiple print heads with colored binder, allows printing in full color.
Among the variety of powders available, calcium sulfate (CaSO4, Product No. 255696 and 237132) is one of the most widely-used because of its ability to react with water-based binders. It can react with water-based solutions rapidly and change into gypsum (CaSO4 ∙ 2H2O) in a solid state.19 In this method, the binding strength is the key factor in determining the physical and chemical properties of the printed device. Therefore, the proper combination of powder and binder should be carefully considered.
Very recently, Voxeljet developed the world’s largest industrial PBP system (VX4000) for sand molds. The largest cohesive build space is 4,000 × 2,000 × 1,000 mm (L × W × H) with 300 μM of a layer applied in one cycle.20
Stereolithography (SLA)
SLA combines ultraviolet (UV) or visible laser light with curable liquid photopolymer resins. To create each layer, a laser beam illuminates a 2D cross-section of the object in a vat of resin, allowing the resin to solidify. Next, the object is raised by an equal distance of layer thickness to fill resin under and maintain contact with the bottom of the object. This process is repeated until the entire model is completed, at which point the platform is raised out of the vat and the excess resin is drained. Finally, the SLA object is finished by washing and curing under UV light. SLA produces a smoother surface on the final product compared to other 3D printing methods, as a result of using liquid photopolymers. Although SLA can produce a wide variety of shapes, its drawbacks include a significant amount of resin waste and the need for extensive cleaning after fabrication. Furthermore, resins used in the process are limited to either epoxy or acrylic bases, most of which can shrink upon polymerization.
A recent advance in SLA significantly decreases printing time. Carbon 3D Inc. announced a new continuous liquid interface production (CLIP) method that can print an object 100 times faster than existing methods by creating an oxygen depletion zone (dead zone) in liquid resins as shown in Figure 4.21 The introduction of a unique oxygen-permeable window in the resin reservoir creates a thin liquid interface of uncured resin between the window and printing part. This oxygen-depleted dead zone allows for continuous translation and curing of the resin above the dead zone to form a consistent solid part.
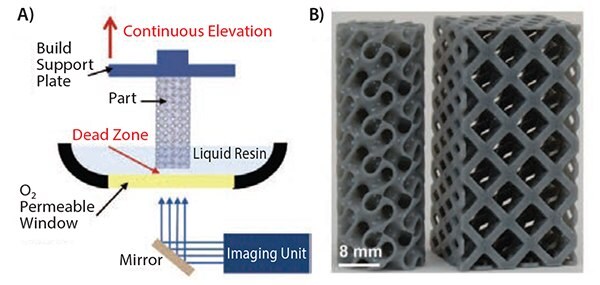
Figure 4.A) Schematic of a CLIP printer. B) The resulting parts via CLIP at print speeds of 500 mm/hour. Copyright 2015, The American Association for the Advancement of Science.
Future Prospects
Three-dimensional printing technology is highly versatile and efficient with respect to design, fabrication, and applications. 4D printing may be of great importance in the future due to its potential to redefine manufacturing-related industries. However, the technology must be further refined before it can replace conventional manufacturing methods. Therefore, future research and investment in 3D and 4D printing technologies are imperative to bring about improvements in essential areas including materials, printer systems, and product markets.
References
Um weiterzulesen, melden Sie sich bitte an oder erstellen ein Konto.
Sie haben kein Konto?