Oxygen & Nitric Oxide Probes
Oxygen Probes
Ruthenium based complexes have been widely used for detection and quantification of oxygen. Fluorescence is strongly quenched by oxygen. Its benefits are:
- Long lifetime and emission around 613 nm minimizing autofluorescence background
- Wide range of published applications
- Ru(dpp)3(PF6) proved to be very useful for use in polystyrene membranes or beads
- Excellent stability and photostability
Fluorogenic Nitric Oxide Imaging Probes
Biological Relevance
Fluorogenic Probes
References
Biological Relevance of NO probes
Nitric oxide (NO) plays a major role as signal transmitter in neurons, endothelial cells and in the immune system. NO has been implicated in vasodilation1, neurotransmission2, cytotoxicity, immune response3 and inflammation4,5,6.
Within cells, nitric oxide synthase (NOS) catalyses the conversion of arginine to citrulline and NO in the presence of molecular oxygen, tetrahydrobiopterin, NADPH and flavin cofactors. Nε-hydroxy-L-arginine is an intermediate in this reaction.
NO has a half-live in vivo of only a few seconds7, however, since it is soluble in both aqueous and lipophilic media, it readily diffuses through the cytoplasm and across cell membranes. NO has effects on neuronal transmission as well as on synaptic plasticity in the central nervous system. In the vasculature NO activates guanylate cyclase, thus increasing the synthesis of c-GMP, which in turn induces smooth muscle relaxation and vasodilation. Another interesting aspect is NO toxicity related to its ability to combine with superoxide anion to form peroxynitrite, an oxidizing free radical that can damage DNA and other cell constituents.
Due to the importance of NO real time detection and quantification of NO is of great interest. As NO is unstable and it’s concentrations in biological systems can be very low, detecting methods with high sensitivities and specificities are required. Imaging of NO concentration based on fluorescence microscopy with its high spatial-temporal resolution seems to be the method of choice provided suitable NO probes are available.
Fluorogenic Probes
In search of a selective NO trapping its transformation with phenylic, vicinal diamines in the presence of molecular oxygen was found to allow the design of sensitive NO probes.8
Weakly fluorescent vicinal diamines can be transformed into their corresponding triazoles by NO and O2, resulting in a strong increase of fluorescence. This mechanism is the basis of most if not all fluorogenic NO probes9. 2,3-Diamino-naphthalene (DAN, 88461) was established as a sensitive probe to measure NO-concentration in vitro and under physiological conditions10. The respective 2,3-naphthotriazole (NAT) was detected in the low nmolar concentration range.

Figure 1.Emission spectra of DAN and NAT at excitation 365 nm (dashed lines) and 375 nm (solid lines) respectively (from reference 9).
However DAN turned out not to be ideally suited to detect NO in cells due to rapid leakage through cell membranes after loading and due to its rather short exitation/emisson wavelengths which cause high background fluorescence in biological matrices. Therefore DAN-1 (and its cell membrane permeable precursor DAN-1 EE were developed8. DAN-1, formed after hydrolysis of DAN-1 EE by cytosolic esterases and its fluorescent reaction product with NO, DAN-1-T showed indeed a considerably improved leakage behavior.
![DAN-1 fluorescence intensity time course depending on [NO] (from ref. 8). An NO releasing reagent (a NONOate) was injected (arrow) into a solution of DAN-1 in buffer pH 7.4 with a final conc. of a) 5 μM and b) 50 μM and the fluorescence intensity measured over time at 440 nm with excitation of 365 nmProbes for real-time detection in living cells. fluor_intens_fig21](/deepweb/assets/sigmaaldrich/marketing/global/images/technical-documents/articles/cell-culture-and-analysis/imaging-analysis-and-live-cell-imaging/fluor_intens_fig21/fluor_intens_fig21.jpg)
Figure 2.DAN-1 fluorescence intensity time course depending on [NO] (from ref. 8). An NO releasing reagent (a NONOate) was injected (arrow) into a solution of DAN-1 in buffer pH 7.4 with a final conc. of a) 5 μM and b) 50 μM and the fluorescence intensity measured over time at 440 nm with excitation of 365 nmProbes for real-time detection in living cells.
Probes for Real-time Detection in Living Cells
DAN-1 however showed disadvantages on real-time detection of NO in living cells such as short exitation/emisson wavelengths, cytotoxicity, strong autofluorescence, small extinction coefficient and unsufficient solubility in neutral buffer.
In order to eliminate those drawbacks DAF-2 and its cell membrane permeable precursor DAF-2-DA have been developed11,12. This probe is based on the widely used fluorescein fluorophore, which combines convenient spectral properties and considerable fluorescence brightness, also in water (see table 1). Having similar extinction coefficients, quantum yield of the probe DAF-2 as such and its NO reaction product DAF-2 T is described to be 0.005 and 0.92 respectively, so that binding of NO results in a ca. 20-fold increase of fluorescence.
The limit of detection of NO by DAF-2 is 5 nM in vitro, i.e. in the absence of absorbing side products. Oxidized forms of NO such as NO2- and NO3-, but also reactive oxygen species, such as O2.-, H2O2, ONOO- do not react with DAF-2 to give a fluorescent product, therefore under physiological conditions DAF-2 T is not formed in the absence of NO.
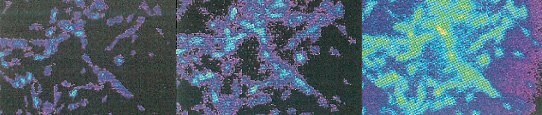
Figure 3.Fluorescence response of cultured, DAF-2 DA loaded smooth aortic rat muscle cells.
- endotoxin- and cytokine-activated cells, with 1 nM L-Arg.
- endotoxin- and cytokine-activated cells, with 1 nM L-Arg and 10 mM L-NMMA.
- inactivated cells with 1 nM L-Arg
Photostable Rhodamine Based Fluorogenic NO-probes
In spite of its excellent properties, some of the characteristics of DAF-2 are undesirable, such as limited photostablity, overlap of fluorescence with in vivo-autofluorescence and loss of fluorescence in the pH range below about 6. Therefore analogous rhodamines have been developed, namely DAR-1 (Product No. 09014), DAR-2 (Product No. 08715)13,14 with spectroscopic data as shown in table 1.
Spectroscopic properties of selected NO-probes
Data at 20° in 0.1 M phosphate buffer pH 7.4; quantum yields derived by comparison with known quantum yield of rhodamin B.13
Despite the lower quantum efficiencies, the rhodamines show high s/n ratios due to lower background of biological matrices in the probes optimum spectral range. Photostablity of these rhodamines is indeed much better than for DAF-213, and the useful pH range extends down as far as pH 4. The NO reaction products of DAR-1 and DAR-2 showed slight instability of the fluorescence signal around pH 7 which was explained by the pKa value of the triazole proton around 7. Therefore a methylated analog, DAR-M was introduced 13,14 (Table 1 and Figure 4).

Figure 4.pH dependent fluorescence intensity of triazol forms of
* from ref. 12,13,14
** based on fluorescence intensity measurements after exposure to sunlight for 3 h.
References
Um weiterzulesen, melden Sie sich bitte an oder erstellen ein Konto.
Sie haben kein Konto?